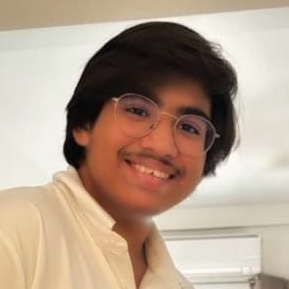
Author: Thrinath Reddy Maddikunta
Mentor: Dr Nageh K. Allam
Sri Chaitanya Ratan Tata Campus Sanathnagar
Introduction
There has been a sharp and dramatic rise in the need for storing large quantities of electrical energy in a compact space in recent decades, affecting the progress of many industries and increasing concern over climate change. As the prominence of nanotechnology is growing in the modern world, we need condensed and long-lasting energies for machines. (Zhang et al., 2023) They can be the decarbonising agents of global energy sectors and most probably make production and society more eco-friendly, ultimately reducing warming. Though there have been multiple trials in achieving more electricity in a concise space, they have not been effective, and it is a pressing problem that needs to be addressed. Consequently, this causes much disruption in sustainable development. (Kumar et al., 2022)
For an urgent spurt in the growth of sustainability and energy problems, we need to outsource the requirement from micro batteries. Specifically, supercapacitors are an essential source due to their abundance and reliability. This can act as an intermediate between the solutions we are looking out for. One possible hindrance can be achieving high-density storage capacity while still increasing the amount of energy stored on a large scale. (Hahn et al., 2017) The proposed work has to be safe, reliable, light, sizeable, resilient to various climatic conditions, and still be able to give a heavy output of electricity with a compressed space in a more structured setup, and the answers to these needs have to be addressed. This review focuses on the advancement in synthesising efficient energy storage capacity in the renewable sector, and the primary objective is to identify key areas for future research. (Zhu et al., 2020)
Several problems need to be addressed, which makes this review essential. Nowadays, commonly used batteries, such as lithium-ion, have limited storage capacity and lower density. A more competent battery is needed for applications where space is limited, which is inflammable in extreme temperatures. On the other hand, high-density batteries are prone to overheating and fire hazards, which have to be dealt with. (Abdisattar et al., 2022) Moreover, this cannot come at the cost of energy conversion losses. Furthermore, self-discharges and degradation shouldn’t limit the usefulness of compact applications. Thermal management, as mentioned before, should be regulated for the heat to dissipate easily without challenging the storage system through thermal runaway, which can cause a potentially catastrophic chain reaction failure. This causes a dependency on material efficiency and electrolytes. (Sung et al., n.d.)
Electrode material constraints will hinder the process. Though new materials such as Silicon and Graphite have shown promising results, Sterling energy, while reducing the size with stability and manufacturability, is quite complicated. Moreover, a very high-performing battery is demanded by complex machines, which can be environmentally damaging and cause depletion of rare metals. On top of this, the cost of production is a burden to make it a socially acceptable source. Integrating such storage capacity with solar equipment is another complicated process. (Kumar et al., 2022)
Inconsistent energy supply due to weather conditions can degrade the lifetime of the battery, and miniaturising power electronics can reduce the efficiency of solar appliances. Along with performance loss, sustainable materials have to be used to make nature-healthy disposal. Without heavy research and review, the development, technique, material acquisition, and emerging from an adoption stage can damage potential solutions and research directions. For example, solid-state batteries, flow batteries, nano-materials, lithium-sulphur batteries, lithium-air batteries, advanced power conversion thermal management, and many more concepts that are in a fundamental stage can give a promising result when compared to traditional techniques, if proper research is done. (Zhang et al., 2023)
This aspect is important, as such technologies can be used for grid stability and energy securities if not in the mainstream resources. Moreover, remote areas can benefit from efficient storage and can acquire a consistent power supply without dependent energy, such as fossil fuels, leading to greater economic activities and a better quality of life. Subsequently, there will be greater energy autonomy and compatibility with advanced technologies, as good storage techniques can perform better and provide larger amounts of electricity in a single unit. Over and above this, science and industry will benefit the most. (Kumar et al., 2022)
Innovative materials and technologies using electrochemistry can foster and push the boundaries of innovations. Besides integrating with emerging technologies, there will be a reduction in operational costs and an improved supply chain of essentials. What’s more, the public will get access to electronic vehicles and urban air movement. Furthermore, microgrid infrastructure and solutions can create energy independence on a large scale. The most important objective of earth, SDGs, will be fulfilled. Research in this area can act as a catalyst for smart cities, extraterrestrial explorations, and the creation of a new and competitive global market. (Hahn et al., 2017) Ultimately, there will be a circular economy that can lower carbon emissions and increase the productivity scale. This can only be done through deep research and reviews of the various aspects involved in this area. Therefore, in this review, we will focus on the ways to improve energy storage to further deepen the understanding of the developments and required changes to excel in this field of study and execution, specifically on the need for integration of batteries with supercapacitors to create microsized energy producers which can survive any conditions. (Abdisattar et al., 2022)
Overview of Technology Involved
Before assessing the research data regarding micro supercapatteries, we need to define a few terms and their prominence and the problems associated with this equipment in the industry of electronics. Micro supercapacitors are carbon and metal oxide-based conducting polymers which have high power density and long life spans. Their energy storage mechanism makes them an essential aspect in addressing the problems previously mentioned. (Sung et al., n.d.) Moreover, MSCs can be easily scaled into various purposes such as laser scribing, printing, and other aggressively integrated circuits which require microscale apparatus. Moreover, the Electric Double Layer Capacitance helps charge and discharge at a very high rate without any chemical reactions. MSCs can even shift from high to medium and low energy densities according to the purpose. This aspect is important in solving the problem of inferno and dangerous reactions caused by thermally active electrolytes. Along with this, their division into aqueous, organic, and solid electrolytes helps them become a versatile tool in every industry. (Abdisattar et al., 2022)
MSCs have become more prominent due to the rise of edge computing and microprocessors by reducing their latency in the network age. Along with this, placing microchips on the MSCs itself makes it far easier to improve the power efficiency of low-power devices. In this process, the competence of high-frequency devices and data centres also improves. One of the top issues addressed is the resistance towards any environmental condition. On top of this, Biomedical, Hybrid systems, microscale robots, rapid response, flexible electronics, IoT, and miniature electronics have caused a huge improvement in the technology. (Zhang et al., 2023) Micro Supercapacitors exhibit lower density, thus making it harder for them to fit along with micro batteries. They even have high discharge rates. Their cost of fabrication and complex manufacturing techniques create voltage limitations and can have high maintenance costs due to the materials used in fabrication. Heat dissipation is another problem which integrates with packaging challenges, electrochemical disproportions, and mechanical stability. What’s more, the most important problem of miniaturising the system can be at peril due to the instability caused by the electrolytes, leading to environmental havoc. (Zhu et al., 2020) One more electronic object which is considered equally important by this paper is Micro batteries. Micro batteries are nano-scaled battery storage devices capable of providing electricity to minute electrical appliances.
To solve the problem at hand, micro-batteries should also be able to store huge amounts of electricity in a minute space. Different types of micro-batteries, such as thin-film batteries, solid state, micro, and 3D-microbatteries, can help us in finding solutions. (Ni et al., 2020) These are generally made of carbon-based materials as electrodes and other polymer-based electrolytes to prevent leakage in the compact setup. These batteries are highly sought after for their usage in medical equipment, energy harvesting devices, and microelectromechanical systems (MEMS). Their integration into nanotechnology to significantly boost macro-devices has made microbatteries a prominent subject. In the age of the rise of wearable technologies, micro-batteries promise a flexible device. Moreover, they can help create self-sustained harvesting systems in the renewable sector. (Sung et al., n.d.)
Micro batteries’ low energy density makes them incapable of attending to the needs of high-energy applications. They degrade and discharge quickly under unfavourable conditions. In the process of diminishing these problems, they face fabrication complexity, making it harder to produce a large- scale commercial stock. These batteries even face hindrances through the instability of the electrolyte in compact spaces. Using micro-batteries without interfering with the functionality of a device is impossible, making them both the energy providers and drainers. Micro-batteries can even frequently cause thermal and electrical hazards. (Kumar et al., 2022)
Integration
Combination of Micro-Super-Capacitors and Micro-Batteries
The combination of energy density and power density helps research plunge into a new application of devices with heavy fluctuating power needs. The blending of their advantages can have dramatic effects. Specifically in electric vehicles and devices, the demand can be supplied, and peak loads can be maintained without taxing the energy drain that generally occurs with micro batteries. Additionally, satellite communication can be a lot easier due to the advancement of CubeSat and MicroSatellites, which use this hybrid technology. (Ni et al., 2020) Likewise, the rovers and landers on other exo-bodies will be able to continue working in extremely harsh climates without any hindrance. Over and above, these can be imitated by the military sector, enhancing the defence capabilities. For example, the flight time of drones can increase drastically, and wearable technology, along with other tactical electronics, can weigh highly lighter and work more efficiently due to this technology. More importantly, these are most useful in increasing the capabilities of the solar energy sector due to power storage capacity. (Saqib et al., 2024)
To achieve the ambitions and solve the limitations mentioned in the introduction, a hybrid storage system known as supercapattery combines electrochemical double-layer (EDL) electrodes. Transition metal phosphides play a significant role in this process. Especially those with metalloid properties and superior redox activity are preferred: nickel, iron, zinc and phosphate. (Saqib et al., 2024) For this, there are proposals for a tri-metallic phosphide nanosheet structure. The electrostatic charge adsorption of faradaic reactions of supercapacitors is exploited for this reason. The disadvantages and advantages of monometallic and bimetallic phosphates make space for improvement in performance. (El Sharkawy et al., 2023)
This amalgamation can be highly useful in absorbing excess energy in the renewable sector during peak generation periods. Most importantly, these can be power backup sources in divisions where a continuous supply of energy is essential, such as data centres and hospitals. This cross-breed can also help build machines, from portable consoles and glucose monitors to cranes and excavators. Interactive packaging and electronic labels due to micro equipment can make global trade a lot more convenient and smoother. (Ni et al., 2020) Kinetic charging solar-powered chips help design construction vehicles with a lot more rapid movement. Above these, the technology helps combine Augmented and Virtual Reality. This helps make tracking the environment a lot easier. The most important aspect is the advancement of technology in the agriculture sector. Hybrid storage systems can help power smart agriculture systems, such as autonomous farm equipment, such as high-power navigation. (Saqib et al., 2024)
New research is using solvothermal methods by a synthesis process in which metal salts are dissolved in sodium hypophosphite as the phosphorus source in a mixture of water and dimethyl formamide. Heating them for extended hours at 180 degrees Celsius leads to the formation of trimetallic nanosheets. The key innovation is the involvement of phosphorous vacancies using NaBH4. This creates active sites for reactions at different concentrations. (Ni et al., 2020) These results can be characterised by techniques. For example, Field Emission Scanning Electron Microscopy revealed that pristine vacancies and interconnections ensure high ion and electron transport. Then, X-ray Photoelectron Spectroscopy and Electron Paramagnetic Resonance confirmed the vacancies in reduced samples and increased signal intensity. These results were further confirmed by Energy Dispersive X-ray Spectroscopy (EDX) and Mott-Schottky analysis, which revealed the enhancement of material conductivity. This study reveals the superior nature of this amalgamation over other energy enhancers. (El Sharkawy et al., 2023)
To understand the improvement of battery efficiency of these vacancies, processes such as cyclic voltammetry (CV), galvanostatic charge-discharge (GCD), and electrochemical impedance spectroscopy (EIS) were used. CV revealed the promotion of redox reactions leading to higher capacity. GCD demonstrated the existence of an aerial capacity of 631.8 mC/cm sq. for a density of 1mA/cm sq., which exhibits a double capacity. Such techniques led to the findings of an optimal concentration of 3M and a reduced time of 2 hours for increased electrochemical performance. (Ni et al., 2020) To assess the practical capabilities of this hybrid application, an asymmetric supercapacitor is used by using a trimetallic electrode on the positive side and activated carbon as negative electrode. This combination revealed the retaining nature of energy density even at a high current density. Along with this, the drive maintained excellent long-term stability. Moreover, further analysis revealed that in the electrochemical mechanism, phosphorus vacancies facilitated deeper ion penetration, thus resulting in an enhanced capacity. (El Sharkawy et al., 2023)
Improvements
For this result to be enhanced, improvement in new inventions, such as sub-100 nm oxidised transition metal tubular architectures, is required. These structures are of great interest due to their heavy usage in dye-sensitised solar cells (DSSCs) and metal oxide field-effect transistors (MOSFETs). For example, titanium dioxide, which is sub-100-nm, is widely used as a photoanode material in DSSCs due to its high surface area and light harvesting capabilities. (US20210292183A.Pdf.3, n.d.) The main advantages of the new invention are to make these tubular systems cost-effective and provide superior performance in their one-dimensional structure, where 80-100% of the nanotubes are less than 100 nm in length and hollow and partially crystalline. In this application, transition metals such as zirconium and vanadium are also used due to their primary relevance with energy applications such as DSSCs. (Ismail et al., 2023)
The method of synthesis for these tubes includes oxidising transition metals in fluid mediums consisting of an electrolyte. This is conducted via galvanic anodisation under strict parameters such as reaction time and composition ratio. Annealing enhances capability. This improves light absorption of panels and has greater quantum efficiency, leading to better battery storage capabilities. US20210292183A.Pdf.3, n.d.) Moreover, this application can be used for photoelectric water splitting and field effect transistors along with high-performance lithium-ion batteries. The experiential data on morphology, crystallinity, and electrochemical performance of tubes, and image confirmations from SEM and TEM, XRD and Raman spectroscopy confirmed the hollow tube’s structure and anodised state enhanced energy production. (Ismail et al., 2023)
Material Advancement
For improving the performance of supercapacitors in hybrid applications, hydrogel electrolytes can be used to address common hindrances in energy storage devices. Hydrogel electrolytes are based on lithium bromide and polyvinyl alcohol, which exhibit high ionic conductivity, non-flammability, self-regeneration, and anti-freezing properties, which is exactly the requirement for supercapattery. (Saqib et al., 2024) Due to this electrolyte, traditional supercapacitors will no longer be affected by solid-state fuel, increasing flexibility, water retention, and convenient packaging. Hydrogels are quasi-solid-state electrolytes. Electrolytes of such kind increase the safety of the batteries, prevent water crystallisation, and promote rehydration. Hydrogels are synthesised by dissolving PVA in deionised water and mixing it in various concentrations of LiBr. (US20210292183A.Pdf.3, n.d.)
Techniques such as Differential Scanning Calorimetry, Thermogravimetric Analysis and Potentio- electrochemical impedance spectroscopy are used to test the quality of the product. Due to these electrolytes, products can recover up to 70% of their water, resist freezing till -30 degrees Celsius, and exhibit a high energy density of 20.4 W h kg-1 and a power density of 3430 W kg-1 while continuing to be non-flammable. (Ismail et al., 2023) These results demonstrate efficiency, such as stable self-discharge for 10,800 seconds and pseudocapacitance due to stable discharge. Further, the device can retain 87.8% of its resistance even after 3000 cycles. Density Functional Theory revealed that LiBr binds strongly with water molecules, preventing crystallisation and enhancing water retention. This causes an interaction between PVA and the chemicals, leading to mechanical robustness and ionic conductivity of hydrogel. (US20210292183A.Pdf.3, n.d.)
Sr-doped TiO2 nanotubes can be synthesised through the anodisation of titanium foil in the presence of Sr(OH)2. Due to this, there is a huge change in the morphology of nanotubes in length and quality, thus increasing their help in supercapacitors. SEM, XRD, and XPS have confirmed the Sr-doping to hold structural integrity. Sr-doping enhances the performance of TiO2 nanotubes significantly, offering a potential strategy for electrolyte sub-modeling. (Ismail et al., 2023)
Along with this, lithium-ion batteries (LIBs) and layered transition metal oxides such as LiCoO2, spinels, and polyanionic compounds help in thermal stability, which is an important factor to address. This combination even evades safety concerns of high voltage and capacity fade or structural degradation. (N. Mohamed & Allam, 2020) Precautionary measures such as surface coating and nanostructured cathode doping design can help improve the performance. Though it has a high capacity, LiCoO2 decreases when cycled beyond 4.2V. This can be alleviated by Al2O3 and ZrO2. This event promises improved LIB performance. Further improvement can be expected from layered transition metal oxides such as LiNiO2, LiMnO2, and LiCo1-xNixO2. This combination even reduces the cost. (N. Mohamed & Allam, 2020)
Fresh Methods in the Industry
New methods suggest cyclic stability of molybdenum disulfide by incorporating nitrogen-doped carbon quantum dots, which can help in the enhancement of the supercapattery performance. (N. Mohamed & Allam, 2020) The hybrid of these materials provides high performance even in compact and macro spaces, making this a promising endeavour in advanced energy storage systems. This makes charge and discharge cycles less exhaustive for the minute spaces occupied by micro supercapattery. Moreover, their collaborative nature with nanomaterials makes them even more useful. (Allam & Grimes, 2011)
The main point of this incorporation of N-CQDs in MoS2 is to improve conductivity and remove cyclic distortions. Moreover, N-CQDs enhance electrochemical activity by offering transport to active sites of charge storage in the MoS2 matrix. This brings a composite high capacitance due to the synergistic effect of surface area and high conductance. (El-Deen et al., n.d.) This makes prolonged charging-discharging cycles more pristine. More importantly, N-CQDs maintain the structural integrity of MoS2, which makes making a piece of micro-designed equipment even easier. This composite holds greater value when it sustains thermal run-away. (Sharkawy et al., n.d.) There is a wide range of permutations and combinations possible in this sector. For example, TiO2 nanotube and nanowire arrays can be used to synthesise high-ordered stability and adsorption activities. Moreover, hetero-junction regions can produce more quantum yields of better applications. This versatile nature can be improved in the lithography method.(Saleh, Ahmed, et al., n.d.) On screening the hybrid electrodes of micro-supercapacitors and micro-batteries, with the help of Ag, we can construct a functional and structurally symmetric micro supercapattery that can stay stable for 15,00 cycles with a stability of 2M, even with a simple electrolyte such as H3PO4. Though this can be swayed by fabrication methods, this is better than relying on pseudo supercapacitors in the interface of the solutions. (A. M. Mohamed et al., n.d.)
Recent High-Performances
Ni-co layered double hydroxide nanocubes, zeolitic imidazolate frameworks, bimetallic and ternary metal phosphides, each contributing a unique advantage to the Micro Supercapattery. One of the promising studies explores the use of 3D hierarchical Ni-co layered double hydroxide (LDH) anchored on Co3O4 nanocubes with spongy graphene electrodes to construct high-performance asymmetric supercapacitors (ASC). (Saleh, Ahmed, et al., n.d.) This device can reach a remarkable specific capacitance of 1866 F/g and an energy density of 66.7 Wh/kg at a power density of 800 W/ kg. This superior performance is attributed to the synergetic structure, promoting rapid ion diffusion and active site accessibility, improving both energy and stability. It was able to maintain 90.5% capacitance retention over 10,500 cycles, giving rise to the possibility of a scalable supercapacitor. (El-Deen et al., n.d.)
Moreover, Zeolitic imidazolate frameworks attract considerable tunable porosity. Though these suffer from poor conductivity, their performance can be spurted through the integration of carbon materials such as reduced graphene oxide and converting it into cobalt oxides and phosphides. (A. M. Mohamed et al., n.d.) By pyrolysing ZIF-67 into these derivative materials, specific capacitance is demonstrated, and the derived cobalt oxides exhibit specific capacitance and rate capability in Micro Supercapatteries. The phosphide and selenide compounds perform well with hybrid devices, combining faradaic charge storage. (Saleh, Amer, et al., n.d.)
Another critical advancement includes plasma-induced defect engineering of bimetallic Ni-Co phosphides for supercapattery applications. The integration of phosphidation and defect engineering in one plasma treatment enhanced the performance of Ni-Co carbonate hydroxide, significantly improving redox-active sites. (Allam & Grimes, 2011) The optimised bimetallic oxyphosphate electrode achieved a specific capacity of 1462 C/g. One of the highest. Additionally, a hybrid supercapacitor incorporating these materials delivered 48 Wh/kg at a power density of 800 W/kg, outperforming most Ni-Co-based devices. The synergy between defect creation and phosphidation underscores the potential of plasma treatment in developing next-generation supercapacitor materials. (Saleh, Ahmed, et al., n.d.)
Lastly, fabricating Mn-Ni-Co phosphide electrodes via electrodeposition and low-temperature plasma treatment. These exhibit superior electrochemical performance, achieving a high specific capacity of 1690 C/g in a three-electrode system. (A. M. Mohamed et al., n.d.) The hybrid device, combining three different materials, delivered an energy density of 55.2 Wh/kg and demonstrated excellent retention till 8,000 cycles. This technique yielded significant improvements in conductivity, energy density, and cycling density. (N. Mohamed & Allam, 2020)
Conclusions
The studies collectively emphasise the importance of material design and engineering in advancing the performance of supercapacitors. By leveraging unique nanostructures, hybrid systems, and deflect engineering, researchers have made significant strides in overcoming the limitations of energy density and cycling stability. New materials and combinations stand as promising candidates for next-generation energy storage devices, offering a balance of high -capacitance, long-term stability, and scalability for real-world applications.
There is a need to find a balance between performance, safety, and cost development of cathode materials. There can be further research into novel materials and improved techniques. The improvement of the structure and stability of these materials, along with new compounds that can offer better performance and safety, are required. More focus is required for application into biomedicine due to reduced voltage requirements. Room-temperature reduction methods can offer strategies for the performance of supercapacitor electrodes. Efficient charge storage should be the primary focus of these outcomes. The successful integration of these nanosheets into a supercapattery device further underscores their potential for practising practical energy storage applications.
The research shows a need for new avenues for the development of high-performance, sustainable energy storage devices that can meet the growing demands of portable electronics, electric vehicles, and renewable energy systems. By optimising the concentration of electrolytes, future studies can enhance storage technologies in Microsupercapatteries. A combination of non-flammable and self- generative flexible substances is required. This can potentially revolutionise the industry. This applies to composite chemicals. Low-temperature synthesis methods are required to provide large-scale relatability. All of these requirements, findings, and deficiencies point to some prospects for future research, which can have significant potential for future energy and environmental technologies.
Recommendations
It can be advised that Artificial Intelligence is a must and should be used in trying out various prospective results for a given experiment to save time and money and improve progress, this helps implement various permutations and combinations to predict a result with the help of previous data. Second, electrolytes such as Graphene, which can satisfy all the required needs, have to be employed for research right from the initial stages. Third, Photo-voltaic integration with Supercapatteries should be understood to enable efficiency in the storage and release of energy. Fourth, Quantum capacitance should be worked upon along with Topological Insulators to touch upon the effect of Spintronics on energy storage. Lastly, these should be tried in combinations to enhance the desired macro-natured powerhouse.
References
Abdisattar, A., Yeleuov, M., Daulbayev, C., Askaruly, K., Tolynbekov, A., Taurbekov, A., & Prikhodko, N. (2022). Recent advances and challenges of current collectors for supercapacitors. Electrochemistry Communications, 142. https://doi.org/10.1016/j.elecom.2022.107373
Allam, N. K., & Grimes, C. A. (2011). Electrochemical fabrication of complex copper oxide nanoarchitectures via copper anodisation in aqueous and non-aqueous electrolytes. Materials Letters, 65(12), 1949–1955. https://doi.org/10.1016/j.matlet.2011.03.105
El Sharkawy, H. M., Saleh, A. A., Elbanna, A. M., & Allam, N. K. (2023). NiFeZnP Nanosheets with Enriched Phosphorus Vacancies for Supercapattery Electrodes. ACS Applied Nano Materials, 6(6), 4875–4886. https://doi.org/10.1021/acsanm.3c00606
El-Deen, A. G., Abdel-Sattar, M. K., & Allam, N. K. (n.d.). High-performance solid-state supercapacitor based on Ni-Co layered double hydroxide@Co O nanocubes and spongy graphene electrodes. https://doi.org/./j.apsusc..
Hahn, R., Ferch, M., Hoeppner, K., Queisser, M., Marquardt, K., & Elia, G. A. (2017, July 18). Development of micro batteries based on microfluidic MEMS packaging. Symposium on Design, Test, Integration and Packaging of MEMS/MOEMS, DTIP 2017. https://doi.org/ 10.1109/DTIP.2017.7984497
Ismail, A. A. M., Ghanem, L. G., Akar, A. A., Khedr, G. E., Ramadan, M., Shaheen, B. S., & Allam, N. K. (2023). Novel self-regenerative and non-flammable high-performance hydrogel electrolytes with anti-freeze properties and intrinsic redox activity for energy storage applications. Journal of Materials Chemistry A, 11(30), 16009–16018. https://doi.org/ 10.1039/d3ta02499g
Kumar, N., Kim, S. Bin, Lee, S. Y., & Park, S. J. (2022). Recent Advanced Supercapacitor: A Review of Storage Mechanisms, Electrode Materials, Modification, and Perspectives. In Nanomaterials (Vol. 12, Issue 20). MDPI. https://doi.org/10.3390/nano12203708
Mohamed, A. M., Ramadan, M., & Allam, N. K. (n.d.). Recent advances on zeolitic imidazolate-67 metal-organic framework-derived electrode materials for electrochemical supercapacitors. In Journal of Energy Storage. https://www.sciencedirect.com/science/article/abs/pii/ S2352152X20320181
Mohamed, N., & Allam, N. K. (2020). Recent advances in the design of cathode materials for Li- ion batteries. In RSC Advances (Vol. 10, Issue 37, pp. 21662–21685). Royal Society of Chemistry. https://doi.org/10.1039/d0ra03314f
Saleh, A. A., Ahmed, N., Biby, A. H., & Allam, N. K. (n.d.). Supercapattery electrode materials by Design: Plasma-induced defect engineering of bimetallic oxyphosphides for energy storage. https://www.sciencedirect.com/science/article/abs/pii/S0021979721010006
Saleh, A. A., Amer, A., Sayed, D. M., & Allam, N. K. (n.d.). Electrochimica Acta. https:// www.sciencedirect.com/science/article/abs/pii/S0013468621004874
Sharkawy, H. M. El, Dhmees, A. S., Tamman, A. R., Sabagh, S. M. El, Aboushahba, R. M., & Allam, N. K. (n.d.). N-doped carbon quantum dots boost the electrochemical supercapacitive performance and cyclic stability of MoS. In Journal of Energy Storage. https://doi.org/./ j.est..
Sung, J.-H., Kim, S.-J., Jeong, S.-H., Kim, E.-H., & Lee, K.-H. (n.d.). Journal of Power Sources Flexible micro-supercapacitors. https://www.sciencedirect.com/science/article/abs/pii/ S0378775306015795. US20210292183A.pdf.3. (n.d.).
Zhang, J., Gu, M., & Chen, X. (2023). Supercapacitors for renewable energy applications: A review. Micro and Nano Engineering, 21. https://doi.org/10.1016/j.mne.2023.100229
Zhu, Z., Kan, R., Hu, S., He, L., Hong, X., Tang, H., & Luo, W. (2020). Recent Advances in High- Performance Microbatteries: Construction, Application, and Perspective. In Small (Vol. 16, Issue 39). Wiley-VCH Verlag. https://doi.org/10.1002/smll.202003251
Ni, J., Dai, A., Yuan, Y., Li, L., & Lu, J. (2020). Three-Dimensional Microbatteries Beyond Lithium Ion. Matter, 2(6), 1366–1376. https://doi.org/10.1016/j.matt.2020.04.020
Saqib, Q. M., Mannan, A., Noman, M., Chougale, M. Y., Patil, C. S., Ko, Y., Kim, J., Patil, S. R., Yousuf, M., Shaukat, R. A., Jeon, Y. P., Dubal, D., & Bae, J. (2024). Miniaturizing Power: Harnessing Micro-Supercapacitors for advanced micro-electronics. Chemical Engineering Journal, 490, 151857. https://doi.org/10.1016/j.cej.2024.151857
Ke, Q., & Wang, J. (2016). Graphene-based materials for supercapacitor electrodes – A review. Journal of Materiomics, 2(1), 37–54. https://doi.org/10.1016/j.jmat.2016.01.001
Sharma, S., & Chand, P. (2023). Supercapacitor and electrochemical techniques: A brief review. Results in Chemistry, 5, 100885. https://doi.org/10.1016/j.rechem.2023.100885
Wu, B., Harvey, G., Whitbread, H., Li, H., Deng, D., & Xiao, J. (2022). Pushing the Limits of Micro battery Capacity and Energy. In U.S. Department of Energy & Pacific Northwest National Laboratory, Pacific Northwest National Laboratory. https://www.pnnl.gov/main/ publications/external/technical_reports/PNNL-32631.pdf
Liu, Z., Wang, H. I., Narita, A., Chen, Q., Mics, Z., Turchinovich, D., Kläui, M., Bonn, M., & Müllen, K. (2017). Photoswitchable Micro-Supercapacitor based on a DiaryLethene- Graphene composite film. Journal of the American Chemical Society, 139(28), 9443– 9446. https://doi.org/10.1021/jacs.7b04491
About the author
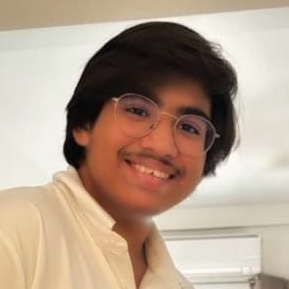
Thrinath Reddy Maddikunta
Thrinath is a 12th-grade student keen to contribute to a sustainable world as an electrical engineer with a current academic focus on the pure sciences. He is passionate about sustainable development and has actively engaged in projects that combine cutting-edge technology with environmental responsibility. Thrinath has designed and implemented IoT-based systems to improve agricultural efficiency, built robotic tools for remote farm management, and is researching advanced energy storage systems through an internship at MIT’s Electrical Engineering and Energy Systems Department with Dr Nageh K. Allam. He is interested in international debates and pursue volunteering, organising, swimming, acting, and Carnatic music, as well as leadership activities in his free time.