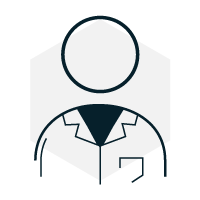
Author: Akshay Gupta
Chattahoochee High School
August 1, 2021
Abstract
With about 285 million visually impaired people worldwide, corneal regeneration is becoming a relevant topic of research among the scientific community. In the last decade, scientists have begun to explore the applications of stem cells to treat various corneal diseases, such as burns, limbal stem cell deficiency, aniridia, and many more. This review paper describes various stem cell therapies for corneal regeneration that are currently being used or researched, with a specific focus on limbal, mesenchymal, and induced-pluripotent stem cell therapies, and provides examples of successful clinical trials supporting the regenerative role of stem cells for corneal repair.
1. Introduction
The cornea is a transparent tissue that constitutes the eye’s outermost lens and acts as a mechanical and chemical barrier that protects the inner ocular tissue (Mansoor et al 2019). The cornea also allows light transmission to the retina (Mansoor et al 2019), and it is estimated that 70% of the eye’s refractive power comes from the cornea. The human adult cornea is about 550 μm thick, and is composed of five layers: epithelium, Bowman’s layer, stroma, Descemet membrane, and endothelium (Eghrari et al 2015). The epithelium is the surface layer and serves as the principal barrier for maintaining fluids in the cornea and for preventing pathogens and bacteria from entering in the deeper layers. The Bowman’s layer posteriorly supports the epithelium and assists in the maintenance of stromal hydration and regulates wound healing by thickening around the wound site (Eghrari et al 2015). The stroma makes up the majority of the cornea’s volume, contributes to clarity, and assists in ocular immunity (Alio Del Barrio et al, 2021). The posterior part of the cornea is composed of the Descemet membrane and the endothelium. Both of these layers are vital for stromal hydration. During embryo development, the cornea first develops with the formation of the epithelium, followed by the production of the stroma and endothelium. Lastly, the Descemet membrane is secreted by the endothelium and the layer thickens (de Oliveria et al, 2020). Currently, there are about 285 million visually impaired people worldwide, with 39 million suffering from blindness. Over 10 million of these cases are caused by corneal diseases that can be caused by traumatic injury, chemical burns, infections, iatrogenesis, age-related degeneration, and corneal dystrophy (Masoor et al 2019). These diseases can cause defects to the cells and the extracellular matrix of the cornea and can cause visual impairment through the formation of corneal scars, haze, and opacities (Mansoor et al 2019), which often lead to blindness if not treated.
The most frequently performed procedure to treat corneal defects is allograft corneal transplantation, which consists in replacing the patient’s damaged cornea with a healthy cornea from a donor. However, this treatment is not ideal as there is limited donor tissue and allograft survival, as well as the need for long-term use of immunosuppressants for patients. Furthermore, many patients cannot get transplants due to the high surgical and rehabilitation costs. Costs of transplantation can cost over $11,000 per year for one person in developed countries. In developing countries, the cost of transplantation can be expected to be much more significant, over double the costs of developed countries (Mansoor et al 2019). Thus, researchers have been looking into corneal treatments based on stem cell technology, as they provide an alternative method to corneal treatment that is not as costly or limited by tissue availability.
2. Stem cell therapies for corneal regeneration
Stem cells are undifferentiated cells that can extensively proliferate and differentiate into different types of cells and tissue (Kolios et al 2013). Advantages of using stem cells therapy for corneal regeneration include the ability to treat a wide spectrum of corneal pathologies, such as blindness and visual impairments (Shukla et al 2019), classical and rare ocular diseases, chemical burns, and limbal stem cell deficiency (Shukla et al 2019). This review paper will cover the most effective stem cell-based treatments developed for cornea regeneration, which exploit the use of limbal, mesenchymal, and adipose-derived stem cells for regenerating damaged or diseased cornea tissues.
2.1. Limbal stem cells
Limbal cells can be found in a narrow zone between the cornea and the bulbar conjunctiva (Rama et al 2010). They maintain homeostasis in the cornea and can cause decreased vision and recurrent epithelial erosions if deficient (Shanbhag et al 2020). Limbal stem cells have the capacity for self-renewal, proliferation, and migration, which make them indispensable for corneal regeneration. Limbal stem cells can be isolated and cultivated in vitro by taking small biopsies of primary cells from human eyes with a minimally invasive procedure (Bremond-Gignac et al, 2018). Researchers have used both autologous cells, i.e. cells isolated from the patient’s healthy eye, and allogeneic cells, that can be isolated from another individual or from a cadaver, for corneal regeneration therapy. Autologous cells are used in cases where only one eye is diseased, and these cells ensure compatibility with the diseased cornea. Allogenic cells are used if both eyes are diseased, but this treatment may require long-term topical or systemic immunosuppressive medication (Bremond-gignac et al, 2018). Limbal stem cells are used mainly for limbal stem cell deficiency (LCSD) and ocular burns (Shanbhag et al 2020). Various techniques are currently investigated to deliver limbal stem-cells to the cornea, which include CLAu (conjunctival-limbal autografting), CLET (cultivated limbal epithelial transplantation), and SLET (simple limbal epithelial transplantation) (Shanbhag et al 2020). CLAu is the traditional method of limbal stem-cell therapy and involves direct transplantation of two conjunctival-limbal lenticules from a healthy eye onto a diseased limbal bed. Autologous CLAu is advantageous as it boasts high efficacy and patients do not need immunosuppressants. CLET is a procedure that consists in taking a 2mm-by-2mm limbal biopsy from a donor eye, isolating and expanding the cells ex-vivo in a clean room for 10-14 days, and then transplanting the grown cells onto the surface of a diseased eye (Bakhtiari et al, 2010). The main advantage of this technique includes the possibility of harvesting a smaller biopsy compared to CLAu, however there are large costs for this procedure and the efficacy is lower than that of CLAu. The SLET method instead is performed by harvesting a strip of donor limbal tissue from a healthy eye, but instead of expanding the cells ex-vivo, the tissue is divided into small pieces and transplanted directly onto a diseased eye for in-vivo expansion. The efficacy of this treatment is similar to that of CLAu and requires a smaller tissue from the donor eye than CLAu. Several clinical studies have shown that limbal stem cell therapy has been proven effective in the treatment of burn-related corneal destruction. In a study from (Rama et al, 2010), renewal of the corneal epithelium was demonstrated in 76% of transplanted limbal stem cells (n=113) delivered on a fibrin substrate with the CLET method. In a study from (Gupta et al 2018), researchers adopted the autologous SLET approach and found that 70% of the treated eyes maintained a successful outcome (n=30). Some disadvantages of LSCT include the dangers of the cultivation process, as allogenic cells can be immunogenic. Furthermore, there is still a lack of a universal and established protocol for limbal stem cell culture in vitro, and the variability of the cell culture conditions among different research groups may influence the outcomes of the clinical trials.
Overall, the studies, Rama et al 2010 and Gupta et al 2018, along with other recent advances in the field demonstrate that limbal stem cells show great potential for corneal regeneration therapies for ocular diseases.
3. Mesenchymal stem cells
Mesenchymal stem cells (MSCs) have been researched as a potential treatment of corneal diseases due to their regeneration and differentiation capabilities. MSCs can be isolated from fetal tissues such as first-trimester blood, bone marrow, placenta, umbilical cord, and amniotic fluid (Mansoor et al 2019). In adults, they can be isolated from bone marrow, peripheral blood, adipose tissue, dermis, synovium, periosteum, cartilage, skeletal muscle, fallopian tubes, menstrual blood, gingiva, and dental tissue (Mansoor et al 2019). MSCs can also be found in the eye, in the corneal stroma and trabecular meshwork (Mansoor et al 2019). Though MSCs can be isolated from a variety of fetal and human tissues, the most typical sources of isolation of MSCs are the bone marrow and the adipose tissue. One way bone marrow MSCs are isolated is through plastic-adhesion. This technique consists of collecting MSCs by specific bone marrow aspiration and selected by their different kinetics of adhesion to the tissue culture vessels (Chu et al 2020). This method is simple and convenient for most researchers, Though effective, extracting the bone marrow from the iliac crest of patients can result in pain, bleeding, or infection, which can make it problematic (Fridman et al 2018). Recently, researchers have been looking for alternatives to bone marrow extraction, which has resulted in MSCs being derived from adipose tissue. These can be more safely and easily isolated, usually resulting in higher yields of isolation (Strioga et al 2012). To isolate adipose tissue MSCs, subcutaneous adipose tissue is used in the form of lipo-aspirates or larger tissue pieces harvested during orthopedic surgery. The mesenchymal stem cells are extracted by finely chopping the tissue and putting it in an enzymatic solution to digest the extracellular matrix, followed by culturing the cells. on culture dishes. (Mushahary et al 2018). MSCs can be isolated also from the cord lining of the human umbilical cord, by dissecting Wharton’s jelly (Mushahary et al 2018). These cells can be used to promote tissue repair and modulate immune responses and anticancer properties (Ding et al 2015). Advantages of fetal-derived MSCs include their longer, more active telomeres and greater proliferation capacity. However, these cells require rigorous ex vivo expansion in order to be used (Mansoor et al 2019). Most studies using MSCs for corneal regeneration have used allogeneic cells. So far, only bone-marrow derived MSCs have been utilized for corneal regeneration purposes, and they have shown to improve corneal re-epithelization. Allogenic bone-marrow derived MSCs have been used to treat limbal stem cell deficiency, in a clinical study performed by Calonge et al (2019). These cells were transplanted through surgery in the cornea. Out of 17 cases given MSC treatment, 85.7% were successful 12 months after surgery (Calonge et al 2019).
MSC treatment can be also administered systemically, using intravenous or intraperitoneal injections, to improve clinical signs in eyes (Galindo et al 2021). A key aspect of fMSCs use for corneal treatments is related to their anti-inflammatory properties, as it has been demonstrated that these cells produce minimal inflammatory response in corneal mechanical damage models (Galindo et al 2021).
Besides being used for transplantation in diseased eyes or for systemic infusion, MSCs can be also cultured in vitro for producing exosomes, unique vesicles containing proteins and genetic information that can be used for regenerative medicine. MSC-derived exosomes can repair tissue damage, suppress inflammatory responses, and modulate the immune system. In addition, exosomes are more stable and reliable, present no risk of aneuploidy (presence of an abnormal number of chromosomes in a cell), and lower chances of immune rejection compared to MSCs. While the mechanisms of exosomes regenerative function are still controversial and not well-understood (Yu et al 2014), they are widely studied for corneal regeneration, as they can be involved in regulating tissue wound repair, inflammation, angiogenesis, and immune response.
4. Induced pluripotent stem cells
Through future research, corneal regeneration may be possible using induced pluripotent stem cells (iPSC). The ability to reprogram somatic cells into iPSCs offers a chance to generate pluripotent patient-specific cell lines that can be used for a variety of diseases (Malik et al 2013). The original way of generating iPSCs was through reprogramming adult human dermal fibroblasts. Through modifying the reprogramming factor expression vectors and experimenting with new modes of delivery, researchers are finding ways to increase efficiency and efficacy of this treatment method. Since iPSCs are easily expandable, they are considered as an unlimited and renewable cell source (Ljubimov et al 2015). However, the current hurdle for corneal regeneration using these cells relates to the optimization of the differentiation of these cells (Ljubimov et al 2015). Currently, iPSCs have been differentiated into limbal epithelial cells using feeder cells, embryoid bodies, and the stromal cell-derived inducing activity method (Zhu et al 2010). But these methods are not yet optimized, which means iPSCs must be further tested and refined for cornea treatments. To the best of our knowledge, there is still no clinical trial using iPSCs for the regeneration and repair of the cornea. However, on the overall, these stem cells can constitute a robust and promising resource for cornea treatments.
5. Conclusion
Currently, there are about 285 million visually impaired people worldwide, with 39 million suffering from blindness. Over 10 million of these cases are caused by corneal diseases (Mansoor et al). To combat this, scientists have been researching ways for corneal regeneration. An innovative and interesting way for corneal regeneration is being researched in the use of stem cells to treat various corneal problems. The use of stem cells like limbal and mesenchymal stem cells have shown great promise in clinical studies. In addition to these, induced pluripotent stem cells are potential candidates for further advancing the field of cornea regeneration as it is a unique and innovative treatment. Therefore, there is no doubt in the recent progress in stem cell treatment for the cornea and the viability of stem cell treatments for corneal diseases in the near future.
References
Alió Del Barrio JL, Arnalich-Montiel F, De Miguel MP, El Zarif M, Alió JL. Corneal stroma regeneration: Preclinical studies. Exp Eye Res. 2021 Jan;202:108314.
Berebichez-Fridman R, Montero-Olvera PR. Sources and Clinical Applications of Mesenchymal Stem Cells: State-of-the-art review. Sultan Qaboos Univ Med J. 2018 Aug;18(3):e264-e277.
Bakhtiari P, Djalilian A. Update on limbal stem cell transplantation. Middle East Afr J Ophthalmol. 2010 Jan;17(1):9-14.
Bremond-Gignac D, Copin H, Benkhalifa M. Corneal epithelial stem cells for corneal injury. Expert Opin Biol Ther. 2018 Sep;18(9):997-1003.
Calonge M, Pérez I, Galindo S, Nieto-Miguel T, López-Paniagua M, Fernández I, Alberca M, García-Sancho J, Sánchez A, Herreras JM. A proof-of-concept clinical trial using mesenchymal stem cells for the treatment of corneal epithelial stem cell deficiency. Transl Res. 2019 Apr;206:18-40.
Chu DT, Phuong TNT, Tien NLB, Tran DK, Thanh VV, Quang TL, Truong DT, Pham VH, Ngoc VTN, Chu-Dinh T, Kushekhar K. An Update on the Progress of Isolation, Culture, Storage, and Clinical Application of Human Bone Marrow Mesenchymal Stem/Stromal Cells. Int J Mol Sci. 2020 Jan 21;21(3):708.
de Oliveira RC, Wilson SE. Descemet’s membrane development, structure, function and regeneration. Exp Eye Res. 2020 Aug;197:108090.
Ding DC, Chang YH, Shyu WC, Lin SZ. Human umbilical cord mesenchymal stem cells: a new era for stem cell therapy. Cell Transplant. 2015;24(3):339-47.
Eghrari AO, Riazuddin SA, Gottsch JD. Overview of the Cornea: Structure, Function, and Development. Prog Mol Biol Transl Sci. 2015;134:7-23.
Galindo S, de la Mata A, López-Paniagua M, Herreras JM, Pérez I, Calonge M, Nieto-Miguel T. Subconjunctival injection of mesenchymal stem cells for corneal failure due to limbal stem cell deficiency: state of the art. Stem Cell Res Ther. 2021 Jan 13;12(1):60.
Gupta N, Joshi J, Farooqui JH, Mathur U. Results of simple limbal epithelial transplantation in unilateral ocular surface burn. Indian J Ophthalmol. 2018 Jan;66(1):45-52.
Kolios G, Moodley Y. Introduction to stem cells and regenerative medicine. Respiration. 2013;85(1):3-10.
Ljubimov AV, Saghizadeh M. Progress in corneal wound healing. Prog Retin Eye Res. 2015 Nov;49:17-45.
Malik N, Rao MS. A review of the methods for human iPSC derivation. Methods Mol Biol. 2013;997:23-33.
Mansoor H, Ong HS, Riau AK, Stanzel TP, Mehta JS, Yam GH. Current Trends and Future Perspective of Mesenchymal Stem Cells and Exosomes in Corneal Diseases. Int J Mol Sci. 2019 12;20(12):2853.
Mushahary D, Spittler A, Kasper C, Weber V, Charwat V. Isolation, cultivation, and characterization of human mesenchymal stem cells. Cytometry A. 2018 Jan;93(1):19-31.
Rama P, Matuska S, Paganoni G, Spinelli A, De Luca M, Pellegrini G. Limbal stem-cell therapy and long-term corneal regeneration. N Engl J Med. 2010 Jul 8;363(2):147-55.
Shanbhag SS, Nikpoor N, Rao Donthineni P, Singh V, Chodosh J, Basu S. Autologous limbal stem cell transplantation: a systematic review of clinical outcomes with different surgical techniques. Br J Ophthalmol. 2020 Feb;104(2):247-253.
Shukla S, Shanbhag SS, Tavakkoli F, Varma S, Singh V, Basu S. Limbal Epithelial and Mesenchymal Stem Cell Therapy for Corneal Regeneration. Curr Eye Res. 2020 Mar;45(3):265-277.
Strioga M, Viswanathan S, Darinskas A, Slaby O, Michalek J. Same or not the same? Comparison of adipose tissue-derived versus bone marrow-derived mesenchymal stem and stromal cells. Stem Cells Dev. 2012 Sep 20;21(14):2724-52.
Yu B, Zhang X, Li X. Exosomes derived from mesenchymal stem cells. Int J Mol Sci. 2014 Mar 7;15(3):4142-57.
Zhu J, Slevin M, Guo BQ, Zhu SR. Induced pluripotent stem cells as a potential therapeutic source for corneal epithelial stem cells. Int J Ophthalmol. 2018 Dec 18;11(12):2004-2010.
About the author
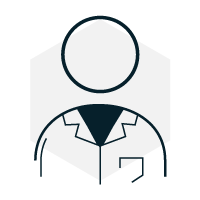
Akshay Gupta
Akshay is a junior at the Chattahoochee high school in Johns Creek, Georgia. He has a strong passion for biology and life sciences. Besides his academic interest in biology, he plays competitive tennis and enjoys working with computers, whether it is building a PC or playing video games.