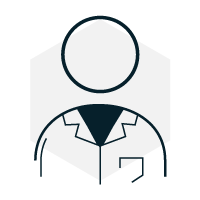
Author: Jhanavi Yogesh
Mentor: Dr. Hong Pan
PES University
Abstract
CRISPR/Cas9 genome editing has undergone a significant transformation that has made it simple, quick, and more accurate for use in immune system and plant applications. CRISPR/Cas9 in plants aids in the development of transgene-free crops with better characteristics, such as greater yields and tolerance to environmental stress and illnesses. Even more exact modifications to the DNA are possible because of new methods like base editing and prime editing, which lower the possibility of unwanted consequences. Furthermore, precision is increased by ribonucleoprotein (RNP)-based editing since no unnecessary DNA is introduced. CRISPR/dCas9 is used in immunology for epigenome editing, which affects the expression of genes without changing the DNA itself. This is very helpful for maintaining the stability of Foxp3, in T cells, which regulates the immune system. Overall, these developments help in building more resilient crops and new treatments for diseases, highlighting the potential of CRISPR technology across various fields of biology.
Key terms:
CRISPR/Cas9 – A powerful tool that scientists use to edit DNA in living things. It works like a pair of scissors, allowing researchers to cut DNA at specific places to make changes.
Genome Editing- The process of making changes in the DNA of an organism to improve its characteristics.
Epigenome Editing- To change how genes are turned on or off without changing the DNA itself. Helps to keep track of how genes behave in cells.
Ribonucleoprotein (RNP)- A combination of RNA and proteins that is used in CRISPR editing to target and modify genes without adding new DNA to the organism’s genome.
Off-Target Effects – Changes to the DNA that occur at places other than where scientists wanted to make edits, leading to unexpected results.
Foxp3- This gene helps in controlling the immune system, required for proper functioning of T regulatory cells.
dCas9 (Dead Cas9)- It is modified Cas9 which binds to the DNA but does not cut it , also used to control the gene activity without changing the DNA.
1.Introduction
During the COVID-19 lockdown in 2020, I watched a Netflix documentary series called Human Nature, which shed light on the significant role of CRISPR technology. As a groundbreaking tool in gene editing, CRISPR has revolutionized genetic engineering by allowing precise alterations in the genome. It offers the ability to correct genetic flaws, introduce beneficial traits, and potentially enhance aspects such as health, intelligence, and appearance. Moreover, CRISPR has opened new possibilities for treating previously incurable genetic disorders, bringing hope to many. The technology also presents the potential for editing the genomes of embryos, which has sparked conversations about its future use in human trait enhancement from the earliest stages of development. Emmanuelle Charpentier and Jennifer Doudna won the 2020 Nobel Prize in Chemistry for their discovery of CRISPR/Cas9, a genome editing technique that allows researchers to precisely change DNA in animals, plants, and microorganisms.
CRISPR/Cas9 is a major advancement over previous genome editing methods such as ZFNs and TALENs. One of its main benefits is the simplicity in designing and implementing guide RNA (gRNA) sequences, which can quickly target specific DNA sites. In comparison, ZFNs and TALENs require intricate protein engineering, making their development more time-consuming and expensive. Furthermore, CRISPR allows researchers to target multiple genes simultaneously, making multi-gene edits more efficient, while ZFNs and TALENs generally focus on one gene at a time. CRISPR/Cas9 also boasts higher editing efficiency and fewer off-target effects, establishing it as a groundbreaking tool in genetic research and biotechnology.
1.1 What is CRISPR? & How CRISPR works
CRISPR/Cas9 is a powerful and flexible tool of gene editing that allows scientists to modify DNA in plants, animals, humans etc. This was first identified as a naturally occurring defense refrain in bacteria. By interacting with the guide RNA, Cas9 is directed to specific DNA sequences and creates double-strand breaks there. This allows scientists to knock out or silence genes and also introduce new desired genes there by providing a vast option in fields such as gene therapy, agriculture, biotechnology etc. All of this happening in such a straightforward, quick manner makes it now the most common technique used today for genetic research and medicine(Koonin et al., 2023)(Okada et al., 2017).
The steps on how CRISPR works is as follows:
- Identification of target sequence
- Guide RNA Design: The first step involves designing a guide RNA (gRNA) that matches the specific DNA sequence that needs to be edited. This gRNA directs the Cas9 protein to the target DNA location (Thakore et al., 2016).
- Binding to Target DNA: Once this is inside the cell, the guide RNA leads the Cas9 protein to the desired DNA sequence by matching to its complementary base pairs of the target sequence (Thakore et al., 2016).
- DNA Cleavage: The Cas9 protein then makes a double-strand break at the target site in the DNA (Okada et al., 2017).
- DNA Repair: The cell naturally tries to repair this break. During this process, scientists can either delete/silence the gene or introduce a new desired gene, leading to a genetic change (Okada et al., 2017).

Diagram showing the CRISPR-Cas9 gene editing process Cas9 protein with a guide RNA sequence (sgRNA) that targets specific portion on DNA (blue) At the targeted site, Cas9 creates cuts on DNA that leads to deleting a specific gene or inserting the new gene and thus modifies genetic sequence. One of its uses is the powerful ability it provides to scientists for precision gene editing (for research or therapeutic applications).

This illustration depicts various CRISPR-Cas-based detection methods. These methods utilized the collateral cleavage activity of Cas13 or Cas12 enzymes to detect specific nucleic acid sequences. Which is detected through different methods including fluorescence, colour changes, lateral flow, and electronic signals. These approaches are highly useful for diagnostics, research, and other applications.
1.2 What is Immunity? CRISPR’s Role
Immunity, It refers to the body’s ability to defend itself from the harmful invading viruses, bacteria etc. CRISPR technology is derived from a bacterial immune system which helps
bacteria in defense against viral infections by integrating viral DNA into its genome. The next time the same virus returns, with this knowledge of how to fight it in hand, bacteria can identify and slice up any viral DNA thus disabling its potential for infection. It is this defense mechanism which scientists used to create CRISPR/Cas9, a gene-editing tool used in labs around the world on everything from humans (Thakore et al., 2016). In humans, the immune system depends on Regulatory T cells (Tregs) to manage immune responses and prevent autoimmunity. These cells ensure that the immune system does not attack the body’s own tissues, greatly by expressing the Foxp3 gene, which is crucial for maintaining their role in suppressing harmful immune responses (Okada et al., 2017).
1.3 What is Epigenomics?
Epigenomics is the study of chemical changes to DNA and histone proteins that influence gene expression without altering the DNA sequence itself. These modifications include DNA methylation and histone acetylation or methylation, which affects the chromatin structure and figures out whether specific genes are activated or not. These modifications help scientists understand and further investigate how gene expression is regulated across different cell types and how any disruptions in these processes can lead to diseases like cancer (Thakore et al., 2016). Scientists use tools such as CRISPR/dCas9 and other technologies to accurately modify these epigenetic markers at precise genomic locations, enabling them to explore how alterations in the epigenome impact gene expression (Thakore et al., 2016). Also, epigenome editing involves modifying DNA methylation and histone acetylation to control gene activity, without changing the genetic code itself. Techniques like CRISPR/dCas9 allows scientists to target specific genome sections and adjust these modifications, offering new potential in studying gene regulation and developing treatments by reprogramming cell function (Okada et al., 2017).

This illustration shows how scientists can manipulate gene activity by adding special proteins to specific DNA sequences. These proteins can either activate or deactivate genes by altering the packaging of the DNA within the cell. These techniques are important for studying gene function, cell behavior, and disease mechanisms. Scientists can also employ these methods to screen numerous genes simultaneously or to engineer novel systems that regulate gene expression.

This illustration shows the two main approaches CRISPR-dCas9 used to regulate the Foxp3 gene. In the first approach, using dCas9-TET1, targets the Foxp3 enhancer region (CNS2) to remove DNA methylation. The second approach, modifications to the Foxp3 promoter by adding acetylation marks to histones, activating the gene using dCas9-p300. Both methods aim to stabilize Foxp3 expression in regulatory T cells.

This illustration shows how CRISPR/Cas9 targets specific DNA sequences in plant cells. The guide RNA (gRNA) directs Cas9 to a specific region on the DNA, where it creates cuts in the double strand. To which scientists can add desired genes or delete/ silenced. The plant’s repair mechanism mends the breaks. This way specific characteristics of plants are enhanced.

This illustration shows the beneficial effects of CRISPR-Cas9 genome editing in plants in drought conditions. By removing/deleting drought-sensitive genes and adding drought-tolerant genes with the help of CRISPR-Cas9 improves enzyme balance, defense mechanisms, protein regulation, nutrient uptake and helping plants survive in stressful environments.
1.4 Applications
CRISPR/Cas9 is aiding farmers in cultivating enhanced crops. For instance, scientists have developed disease-resistant wheat, drought-tolerant rice, and cold-resistant tomatoes using this technology. These innovations are essential for helping farmers boost food production, particularly in challenging environmental conditions. However, there are still hurdles to overcome, such as ensuring the safety of these changes and addressing public concerns about the consumption of genetically modified crops (Koonin et al., 2023).
In their research, scientists explored two methods to improve the stability of the Foxp3 gene. The first approach involved using a variant of dCas9 fused with TET1, which removes methylation marks from the DNA in a specific region known as CNS2. While this aimed to activate the gene, it had limited effectiveness.(Okada et al., 2017)
The second approach linked dCas9 to p300, an enzyme that adds acetyl groups to the proteins around the DNA at the Foxp3 promoter. This method proved to be significantly more effective, greatly enhancing the gene’s activity even under stress. As a result, the cells were better able to maintain Foxp3 levels and function effectively, even in difficult conditions (Okada et al., 2017).
1.5 Advances and Challenges in CRISPR/Cas9 Technology
Although CRISPR/Cas9 is a powerful tool, it is not without flaws. Sometimes it cuts DNA at unintended locations, which can lead to issues. Researchers are actively developing new methods, such as base editing, that enable more precise and smaller modifications. In the future, CRISPR could be applied to a wider variety of plants, but challenges remain, including legal regulations and environmental safety concerns (Koonin et al., 2023).
The dCas9-p300 method has shown good results, but there are challenges in maintaining gene expression, particularly during inflammation. Scientists aim to enhance these tools to treat diseases related to immune system dysfunction, like autoimmune disorders (Okada et al., 2017).
While CRISPR/Cas9 is an impressive technology, it can also produce unintended changes, known as off-target effects. Researchers are working to improve its accuracy with techniques like base and prime editing. Additionally, there are regulatory challenges and public concerns regarding genetically modified plants. Nonetheless, CRISPR holds significant promise for addressing global issues like food security by developing stronger and more productive crops (Wada et al., 2020).
1.6 Discussion/Conclusion:
CRISPR/Cas9 technology is a game changer in genetic engineering, offering new opportunities in agriculture, immunology etc. In agriculture, it modifies traits like disease resistance and stress tolerance, reducing the time compared to traditional breeding methods and getting better yield.
In immunology, CRISPR/dCas9-based epigenome editing provides innovative treatment options by regulating gene expression without altering the DNA. This is crucial for stabilizing genes like Foxp3, which is essential for immune regulation, potentially leading to improved treatments for immune disorders.
Despite these advancements, challenges such as off-target effects still exist, although newer methods like base and prime editing are minimizing these issues. Ethical and regulatory debates continue to shape CRISPR’s applications in agriculture and healthcare. In summary, CRISPR/Cas9 and its advancements have transformed gene editing across various fields, enhancing food security and treatments for immune-related diseases. Continued innovation in CRISPR technology, along with careful attention to ethical considerations, is vital for its future development.
1.7 References
1. Koonin, E. V., Gootenberg, J. S., & Abudayyeh, O. O. (2023). Discovery of diverse CRISPR-Cas systems and expansion of the genome engineering toolbox. Biochemistry, 62(24), 3465–3487. https://doi.org/10.1021/acs.biochem.3c00159
2.Ishino, Y., Krupovic, M., & Forterre, P. (2018). History of CRISPR-Cas from encounter with a mysterious repeated sequence to genome editing technology. Journal of Bacteriology, 200(7), e00580-17. https://doi.org/10.1128/JB.00580-17
3. Okada, M., Kanamori, M., Someya, K., Nakatsukasa, H., & Yoshimura, A. (2017). Stabilization of Foxp3 expression by CRISPR-dCas9-based epigenome editing in mouse primary T cells. Epigenetics & Chromatin, 10, 24. https://doi.org/10.1186/s13072-017-0129-1
4. Wada, N., Ueta, R., Osakabe, Y., & Osakabe, K. (2020). Precision genome editing in plants: State-of-the-art in CRISPR/Cas9-based genome engineering. BMC Plant Biology, 20(1), 234. https://doi.org/10.1186/s12870-020-02385-5
5. Sami, A., Xue, Z., Tazein, S., Arshad, A., Zhu, Z. H., Chen, Y. P., Hong, Y., Zhu, X. T., & Zhou, K. J. (2021). CRISPR-Cas9-based genetic engineering for crop improvement under drought stress. Bioengineered, 12(1), 5814–5829. https://doi.org/10.1080/21655979.2021.1969831
6. Thakore, P. I., Black, J. B., Hilton, I. B., & Gersbach, C. A. (2016). Editing the epigenome: Technologies for programmable transcription and epigenetic modulation. Nature Methods, 13(2), 127–137. https://doi.org/10.1038/nmeth.3733
About the author
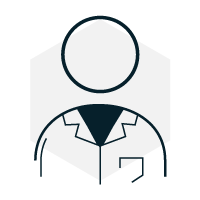
Jhanavi Yogesh
Jhanavi is currently pursuing a B.Tech in Biotechnology at PES University, (India, Karnataka) Bangalore. In addition to interning under Dr. Hong Pan, she has also worked in an IVF lab and completed two internships in advanced bioinformatics at Sciomics, expanding her skills in Python, R programming, and bioinformatics analysis.
Outside of academics, Jhanavi has been an active sportsperson, having held leadership roles as captain in basketball and handball, as well as vice-captain in throwball, and has won medals in swimming relay, long jump, tug of war, and cultural events. She has participated in volleyball at the district level and her passion for sports has helped her develop strong teamwork and leadership skills.