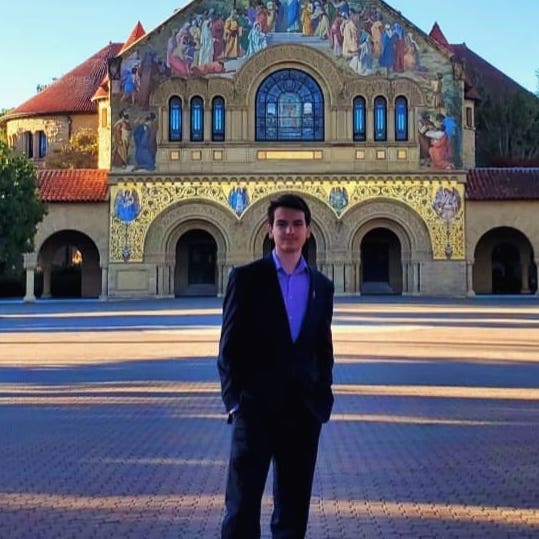
Author: Milutin Todorovic
Mentor: Snezana Tomasevic Todorovic
Glenbrook South High School
Abstract
Osteoporotic vertebral fractures represent a significant sociomedical problem that impairs the quality of life of the elderly population. Clinical examination, additional diagnostic methods (X-ray, CT, DXA, LVA) and fracture risk assessment (Frax) are key for effective assessment of the severity of the fracture, timely decision on the method of treatment, as well as initiation rehabilitation. Of great practical importance is the effect of antiresorptive therapy on formation callus. In patients suffering from osteoporosis, who despite treatment have a fracture, it is recommended not to interrupt bisphosphonate therapy, which was started several months before diagnosed fracture. Bisphosphonates should be introduced into the treatment in a period of 2-4 months from occurrence of the fracture, depending on the location of the fracture, i.e. the time required for callus formation. Recombinant parathyroid hormone is an effective anabolic therapy accelerates bone regeneration during fractures, increases callus volume and faster recovery bone strength. Osteoporosis therapy should not be started without checking the total and ionized Ca, P, 25(OH)D, PTH as well as general biochemical analyses, and then introduce antiresorptive or anabolic therapy. Rehabilitation treatment is designed individually and includes exercises balance, strength, range of motion and postural training lead to improvement spinal mobility, muscle strength and overall functionality. The aim of this review is to emphasize timely diagnosis, evaluation, and treatment of osteoporotic fractures vertebrae.
Keywords: Osteoporotic Vertebral Fractures, DXA, FRAX. Therapy, Rehabilitation
1 Introduction
Osteoporotic vertebral fractures affect approximately 1 in 3 women and 1 in 5 men over the age of 50 (1,2) leaving significant physical, psychological, and social consequences for patients, leading to reduced mobility, functional limitations, and decreased quality of life (3,4). Rehabilitation interventions have been shown to improve pain, functional outcomes, and overall well-being in patients with osteoporotic vertebral fractures (5-7). Pathophysiology and clinical presentation have shown that osteoporotic vertebral fractures result from a combination of reduced bone mineral density and changes in bone microarchitecture (7,8). Studies have demonstrated that the trabecular bone, particularly in the vertebral bodies, is most susceptible to osteoporotic fractures due to its high metabolic activity and abundant remodeling (9-11). Clinically, patients with osteoporotic vertebral fractures may experience acute or chronic back pain, which can be worsened by movement, prolonged sitting, or activities involving spinal loading (12). Height loss and kyphotic deformity are also common clinical features, contributing to postural changes and functional limitations. Potential hip fractures present great potential risk that cannot be overlooked (13- 15).
2 Radiological Examination
Imaging techniques such as X-rays, CT scans, and MRI are used to diagnose and assess the severity of osteoporotic vertebral fractures (7). DXA is the the absolute gold standard (13) for bone mineral density measurements and a widely used technology for assessing bone health and measuring bone mineral density (BMD) (14-16). DXA may offer precise and accurate measurements of BMD at various skeletal sites, including the spine, hip, and forearm. It utilizes low-dose X-ray beams that pass through the bone, allowing for the calculation of BMD based on the differential absorption of these beams by bone and surrounding tissues. The BMD results obtained are compared to reference values, typically provided by age-matched and gender-matched populations, to determine if an individual’s bone density is within the normal range or if osteoporosis or osteopenia is present. In addition, It provides information about body composition. It can determine the percentage of lean mass, fat mass, and total body fat, allowing for the evaluation of body composition changes over time (14-17). This feature is particularly useful in monitoring the effects of interventions, such as exercise and nutrition, on body composition and overall health. Moreover, DXA can estimate the risk of fractures by utilizing algorithms that combine BMD measurements with clinical risk factors. These algorithms, such as the Fracture Risk Assessment Tool (FRAX), provide a comprehensive assessment of an individual’s fracture risk over a specified time period, aiding in treatment decisions and preventive strategies (18). Lateral Vertebral Assessment (LVA) is a radiographic method that provides quantitative measurements of vertebral heights and allows for the identification of vertebral fractures. It offers advantages such as low radiation exposure and quick assessment, making it a valuable tool for screening and monitoring osteoporosis-related fractures. LVA complements DXA by providing additional information about vertebral fractures and aiding in fracture risk assessment (19,20).
3 Fracture risk assessment and risk factors
Factors that increase the risk of developing osteoporosis encompass being 50 years of age or older, being of the female gender, belonging to the Caucasian ethnicity (particularly of northern European or Asian descent), having a genetic predisposition, having a petite and slender physique, experiencing undernourishment, leading a sedentary lifestyle, having a history of amenorrhea, late menarche, or early menopause, suffering from deficiencies in estrogen and androgen hormones, engaging in alcohol consumption or cigarette smoking, maintaining a diet low in calcium, and using certain medications (such as steroids, insulin, anticonvulsants, chemotherapeutics, or heparin) (21-23) The FRAX index combines DXA measurements and clinical risk factors to estimate the probability of future fractures. FRAX integrates clinical risk factors and bone mineral density (BMD) measurements to estimate the 10-year probability of specifically both major osteoporotic fracture (hip, clinical spine, forearm, or shoulder) and hip fracture. The clinical risk factors taken into account by FRAX include age, sex, previous fracture history, parental history of hip fracture, smoking status, alcohol consumption, glucocorticoid use, rheumatoid arthritis, and secondary causes of osteoporosis. By considering these risk factors along with BMD measurements, FRAX provides a comprehensive assessment of an individual’s fracture risk. FRAX calculations are based on large population-based cohorts and validated in numerous studies. It helps identify individuals at high risk of fracture who may benefit from early interventions, such as pharmacological treatments, lifestyle modifications, and fall prevention strategies. FRAX does not consider all possible risk factors, such as vitamin D deficiency or secondary causes of osteoporosis (14-18,24,25).
4 Conservative Management
Conservative management strategies for osteoporotic vertebral fractures aim to alleviate pain, improve function, and promote healing without surgical intervention. Various classes of medications are commonly used for treating osteoporotic fractures. Bisphosphonates, like alendronate and risedronate, inhibit bone resorption (26). Selective Estrogen Receptor Modulators (SERMs), such as raloxifene, mimic estrogen’s effects on bone (27). Teriparatide stimulates bone formation and is administered via injections (28). Denosumab, a monoclonal antibody, targets bone resorption (29). Calcitonin helps regulate calcium levels but has modest fracture risk reduction (29). Due to wide range of pharmacological options today, the complete assessment of the patient overall condition must be made before choosing the appropriate treatment (26-30). When we discuss the efficient medications, we cannot skip to mention that anabolic agents, like romosozumab, promote bone formation by inhibiting sclerostin and are highly effective, especially in postmenopausal women with severe osteoporosis. The effect of antiresorptive therapy on callus formation is important. Bisphosphonates should be introduced into the treatment within a callus formation period which is 2-4 months from the fracture occurrence. Patients who suffer from osteoporosis and despite treatment have a fracture, it is recommended to continue therapy which has started months before the diagnosed fracture.
Recombinant parathyroid hormone is an effective anabolic therapy which accelerates bone regeneration during fractures, increases callus volume and faster the bone strengthening (28,30).
5 Biomarkers
Biochemical markers in bone metabolism, including serum cross-linked C-telopeptide of type I collagen (CTX), N-terminal telopeptide (NTx) ,tartrate-resistant acid phosphatase 5b (TRACP 5b) , and bone-specific alkaline phosphatase (BALP) provide crucial insights into bone health. Elevated CTX and NTX levels indicate increased bone resorption, while higher BALP levels suggest enhanced bone turnover. These markers help assess the effectiveness of treatments like anti-resorptive medications. Additional markers like osteocalcin (OC), serum procollagen type I N-propeptide (PINP), and dihydropyrimidine dehydrogenase (DPD) aid in early bone loss detection and treatment guidance, enhancing patient care when combined with clinical assessments and imaging techniques. Total and ionized calcium Ca, P, 25(OH)D, PTH and general biochemical analyses have to take place before introducing antiresorptive or anabolic therapy (31-33).
6 Rehabilitation of Patients with Osteoporotic Vertebral Fractures
Mechanical stimulation affects bones in two distinct ways. Firstly, repetitive strain can have a negative impact, resulting in minor damage to bone structure. Conversely, strains surpassing a certain threshold stimulate new bone production and enhance bone resilience under greater loads. The interconnectedness of these effects is commonly referred to as the mechanistic theory, which plays a pivotal role in preventing osteoporotic fractures. It revolves around the concept of minimal effective strain (MES), crucial for safeguarding bone density. To maintain bone structure, strain within the physiological range (800-1,500 μstrain) is essential. Deviations from this range yield varying outcomes, including increased bone resorption at less than 800 μstrain, strengthened bones at 1,500–3,000 μstrain, or even pathological fractures at 15,000 μstrain. Hence, rehabilitation programs aimed at preventing bone loss should incorporate kinesitherapy alongside conventional pharmacological treatments (30,33,34). The management of vertebral fractures can be categorized Into three phases: the acute phase, post-acute phase, and the rehabilitation phase. In the acute and post-acute stages, the primary objectives include effective pain management and ensuring the stability of the fracture (35). Conservative treatment involves the use of an orthosis, designed to provide spinal stabilization. Orthoses are typically employed for a duration of 8 to 12 weeks to aid in the healing of fractures. During the initial 8 weeks following a vertebral fracture, patients are advised to refrain from engaging in resistive strength training. However, relaxation exercises, breathing routines, and range of motion exercises can be introduced to counteract joint rigidity (36-40). Still, it is crucial to minimize prolonged bed rest during these phases and instead encourage mobility in patients. Prolonged bed rest has the potential to lead to undesired consequences such as muscle atrophy, weakness, joint stiffness, pressure sores, deep vein thrombosis, respiratory issues, disorientation, and even depression (38,39). Before initiating the rehabilitation phase, radiological assessment of progress in fracture healing is pivotal. In the early stages, it is advisable to introduce neuromuscular stabilization exercises targeting the thoracolumbar region, which help immobilize this area. Within the rehabilitation program, exercises to strengthen the dorsal extensor muscles, aimed at reducing kyphosis, are recommended. Moreover, postural retraining, balance enhancement, and proprioceptive exercises are crucial for minimizing the risk of falls and secondary fractures (39-43). Rehabilitation phase has to be individually tailored, still it must be underlined that the resistance training, balance exercises and postural training lead to improvements in spinal mobility, muscle strength and overall functionality of the patients.
7 Physical Modalities
Physical modalities used to improve bone repair include various therapeutic techniques and treatments aimed at enhancing bone healing and bone health. Some of these modalities include Low-Intensity Pulsed Ultrasound (LIPUS) (44), Electrical Stimulation (45), Functional Electrical Stimulation (FES) (46), Magnetic Field Therapy (47), and Vibration Therapy (48). LIPUS therapy involves the use of low-intensity ultrasound waves to stimulate bone healing. I’’s often used to accelerate fracture healing and can promote the formation of new bone (44). Electrical stimulation methods such as direct current, inductive coupling, and capacitive coupling have been employed to promote bone repair. These modalities can enhance the production of bone cells and help in the healing process (445). FES involves the use of electrical currents to stimulate muscle contractions. I’’s used to counteract muscle atrophy and promote bone health in individuals with limited mobility, such as those with spinal cord injuries (46). Pulsed electromagnetic field (PEMF) therapy uses electromagnetic fields to stimulate bone repair. I’’s often utilized in the treatment of non-union fractures and other bone-related conditions (47). Whole-body vibration therapy and localized vibration therapy are techniques that involve the application of mechanical vibrations to stimulate bone formation and improve bone density (48). It’s important to note that the choice of physical modality depends on the specific condition, the stage of bone healing, and the recommendations of healthcare professionals. Individualized treatment plans are typically created to address the unique needs of each patient.
8 Nutrition in Supporting Bone Healing
Nutritional therapy plays a significant role in supporting the healing process and overall bone health following an osteoporotic vertebral fracture. Calcium is essential for bone health. Ensuring the patient is getting an adequate amount of calcium through dietary sources such as dairy products (low-fat or non-fat milk, yogurt, cheese), fortified plant-based milk alternatives, leafy greens (kale, collard greens), and fortified cereals. Aim should be around 1,000 to 1,200 milligrams of calcium daily, but individual requirements may vary (49). Vitamin D is crucial for calcium absorption and bone health. Spending time in the sun (with sunscreen) to allow body to produce vitamin D naturally. Additionally, including foods rich in vitamin D in diet, such as fatty fish (salmon, mackerel), egg yolks, and fortified foods like orange juice and cereals. Magnesium contributes to bone health by helping to convert vitamin D (50,51) into its active form. Good sources of magnesium include nuts, seeds, whole grains, and green leafy vegetables. Usage of vitamin D supplement if levels are low is mandatory step. Furthermore, Vitamin K is necessary for bone mineralization (52). You can find it in foods like leafy greens (kale, spinach, collard greens), broccoli, Brussels sprouts, and certain vegetable oils. Protein intake is essential for the repair and maintenance of bones and muscles (53). Lean protein sources like poultry, fish, lean meats, beans, lentils, and tofu are preferable. High sodium intake can lead to calcium loss in the urine (54). Maintaining a balanced intake of phosphorus is one of the challenges. While it is important for bone health, an excessive intake can interfere with calcium absorption. Phosphorus is naturally present in many foods, including dairy products, meats, and nuts (55).
9 Conclusion
The comprehensive management of patients with osteoporotic vertebral fractures involves a multidisciplinary approach, combining rehabilitation interventions, radiological examinations, biochemical markers, conservative management strategies, and prevention of complications. By addressing these aspects, healthcare professionals can optimize patient outcomes, alleviate pain, improve functional abilities, and enhance the overall well-being of individuals affected by osteoporotic vertebral fractures.
References
- Sözen T, Özışık L, Başaran NÇ. An overview and management of osteoporosis. Eur J Rheumatol.2017;4(1):46-56.doi: 10.5152/eurjrheum.2016.048.
- Arceo-Mendoza RM, Camacho PM. Postmenopausal osteoporosis: latest guidelines. Endocrinology and Metabolism Clinics. 2021;50(2):167-78.
- Rizzo M, Tammaro G, Guarino A, Basso M, Cozzolino A, Mariconda M. Quality of Life in osteoporotic patients. Orthop Rev (Pavia). 2022;14(6):38562. doi: 10.52965/001c.38562.
- Jung HJ, Park YS, Seo HY, Lee JC, An KC, Kim JH, Shin BJ, Kang TW, Park SY. Quality of Life in Patients with Osteoporotic Vertebral Compression Fractures. J Bone Metab.2017;24(3):187-196.doi:10.11005/jbm.2017.24.3.187.
- Lawrence T. Rehabilitation and Maximizing Function in Long-Term Care. InPostAcute and Long-Term Care Medicine: A Guide for Practitioners. 2023; 369-81
- Imamudeen N, Basheer A, Iqbal AM, Manjila N, Haroon NN, Manjila S. Management of Osteoporosis and Spinal Fractures: Contemporary Guidelines and Evolving Paradigms. Clin Med Res. 2022;20(2):95-106. doi: 10.3121/cmr.2021.1612.
- Tomasević-Todorović S, Simić-Panić D, Knežević A, Demeši-Drljan Č, Marić D, Hanna F. Osteoporosis in patients with stroke: A cross-sectional study. Annals of Indian Academy of Neurology. 2016;19(2):286.
- Griffith JF. Identifying osteoporotic vertebral fracture. Quant Imaging Med Surg. 2015;5(4):592-602. doi: 10.3978/j.issn.2223-4292.2015.08.01.
- Pouresmaeili F, Kamalidehghan B, Kamarehei M, Goh YM. A comprehensive overview on osteoporosis and its risk factors. Ther Clin Risk Manag. 2018;14:2029-2049. doi: 10.2147/TCRM.S138000.
- Liang B, Burley G, Lin S, Shi YC. Osteoporosis pathogenesis and treatment: Existing and emerging avenues. Cellular & Molecular Biology Letters. 2022;27(1):72.
- Wang H, Zhang Y, Ren C, Ding K, Zhang Q, Zhu Y, Chen W. Biomechanical properties and clinical significance of cancellous bone in proximal femur: A review. Injury. 2023 Mar 10.
- Ponzano M, Tibert N, Brien S, Funnell L, Gibbs JC, Keller H, Laprade J, Morin SN, Papaioannou A, Weston Z, Wideman TH. International consensus on the nonpharmacological and non-surgical management of osteoporotic vertebral fractures. Osteoporosis International. 2023;17:1-0.
- Arai T, Fujita H, Maruya K, Morita Y, Asahi R, Ishibasi H. Loss of height predicts fall risk in elderly Japanese: a prospective cohort study. Journal of Bone and Mineral Metabolism. 2023;41(1):88-94.
- Eirini K, Nikolaos T, Papadopoulou SK, Georgios G. Bone Density Measurements and Biomarkers in Nutrition: DXA (Dual X-ray Absorptiometry), Osteopenia, and Osteoporosis. InBiomarkers in Nutrition. 2022; 1067-84.
- Bjelica A, Vučaj ĆV, Tomašević-Todorović S, Filipović K. Postmenopausal osteoporosis. Medicinski pregled. 2018;71(5-6):201-5.
- Haseltine KN, Chukir T, Smith PJ, Jacob JT, Bilezikian JP, Farooki A. Bone Mineral Density: Clinical Relevance and Quantitative Assessment. J Nucl Med. 2021;62(4):446-454. doi: 10.2967/jnumed.120.256180.
- El Maghraoui A, Roux C. DXA scanning in clinical practice. QJM. 2008;101:605–617.
- Silva BC, Leslie WD, Resch H, et al.. Trabecular bone score: a noninvasive analytical method based upon the DXA image. J Bone Miner Res. 2014;29:518–530
- El Miedany Y. FRAX: re-adjust or re-think. Arch Osteoporos. 2020 Sep 28;15(1):150. doi: 10.1007/s11657-020-00827-z.
- Gill SM, Hassan A. Routine Use of Lateral Vertebral Assessment With DXA Scan for Detection of Silent But Debilitating Vertebral Fractures. Clin Nucl Med. 2023;48(2):107-111.Doi:10.1097/RLU.0000000000004494.
- Binkley N, Krueger D, Gangnon R, Genant HK, Drezner MK. Lateral vertebral assessment: a valuable technique to detect clinically significant vertebral fractures. Osteoporos Int. 2005;16(12):1513-8. doi: 10.1007/s00198-005-1891-7.
- Chen YW, Ramsook AH, Coxson HO, Bon J, Reid WD. Prevalence and risk factors for osteoporosis in individuals with COPD: a systematic review and meta-analysis. Chest. 2019;156(6):1092-110.
- Ebeling PR, Nguyen HH, Aleksova J, Vincent AJ, Wong P, Milat F. Secondary osteoporosis. Endocrine Reviews. 2022;43(2):240-313.
- Tomasevic-Todorovic S, Vazic A, Issaka A, Hanna F. Comparative assessment of fracture risk among osteoporosis and osteopenia patients: a cross-sectional study. Open access rheumatology: research and reviews. 2018 May 30:61-6.
- Pantelinac S, Simić-Panić D, Janjić N, Spasojević T, Tomašević-Todorović S. Physical activity and fall prevention-solving clinical problems. Medicinski pregled. 2022;75(Suppl. 2):32-6.
- Drake MT, Clarke BL, Khosla S. Bisphosphonates: mechanism of action and role in clinical practice. Mayo Clin Proc. 2008;83(9):1032-45. doi: 10.4065/83.9.1032.
- Lafront C, Germain L, Weidmann C, Audet-Walsh É. A systematic study of the impact of estrogens and selective estrogen receptor modulators on prostate cancer cell proliferation. Scientific Reports. 2020;10(1):4024.
- Takeuchi Y. How different is the once-weekly teriparatide from the daily one or the same?. Osteoporosis and Sarcopenia. 2019;5(2):27.
- Lim SY. Clinical Application of Monoclonal Antibodies: Key Technological Advances and Treatment of Osteoporosis. Innovative Bioceramics in Translational Medicine II: Surgical Applications. 2022;75-109.
- Tomašević-Todorović S, Ilić N. Contemporary approach to osteosarcopenia. Medicinski pregled. 2022;75(Suppl. 2):68-71.
- Cheng CH, Chen LR, Chen KH. Osteoporosis due to hormone imbalance: an overview of the effects of estrogen deficiency and glucocorticoid overuse on bone turnover. International Journal of Molecular Sciences. 2022;23(3):1376.
- Palui R, Durgia H, Sahoo J, Naik D, Kamalanathan S. Timing of osteoporosis therapies following fracture: the current status. Therapeutic Advances in Endocrinology and Metabolism. 2022; 13:20420188221112904.
- Brown JP, Don-Wauchope A, Douville P, Albert C, Vasikaran SD. Current use of bone turnover markers in the management of osteoporosis. Clin Biochem. 2022;109-110:1-10. doi: 10.1016/j.clinbiochem
- Terreni A, Pezzati P. Biochemical markers in the follow-up of the osteoporotic patients. Clin Cases Miner Bone Metab. 2012;9(2):80-4.
- Kuo TR, Chen CH. Bone biomarker for the clinical assessment of osteoporosis: recent developments and future perspectives. Biomark Res. 2017; 5:18. doi: 10.1186/s40364-017-0097-4.
- Hart NH, Nimphius S, Rantalainen T, Ireland A, Siafarikas A, Newton RU. Mechanical basis of bone strength: influence of bone material, bone structure and muscle action. J Musculoskelet Neuronal Interact. 2017;1;17(3):114-139.
- Song L. Effects of Exercise or Mechanical Stimulation on Bone Development and Bone Repair. Stem Cells Int. 2022; 2022:5372229. doi: 10.1155/2022/5372229.
- Pratelli E, Cinotti I, Pasquetti P. Rehabilitation in osteoporotic vertebral fractures. Clin Cases Miner Bone Metab. 2010;7(1):45-7.
- Tomasevic-Todorovic S , Boskovic K, Čubrilo S. et al. Quality of life in female patients with osteoporotic vertebral fracture. Acta Med Croatica, 71 (2017) 273-78.
- Kweh BTS, Lee HQ, Tan T, Rutges J, Marion T, Tew KS, Bhalla V, Menon S, Oner FC, Fisher C, Tee JW. The Role of Spinal Orthoses in Osteoporotic Vertebral Fractures of the Elderly Population (Age 60 Years or Older): Systematic Review. Global Spine J. 2021 Jul;11(6):975-987. doi: 10.1177/2192568220948036.
- Mak SKD, Accoto D. Review of Current Spinal Robotic Orthoses. Healthcare (Basel). 2021;9(1):70. doi: 10.3390/healthcare9010070 Parry SM, Puthucheary ZA. The impact of extended bed rest on the musculoskeletal system in the critical care environment. Extrem Physiol Med. 2015; 4:16. doi: 10.1186/s13728-015-0036-7.
- Cizza G, Primma S, Csako G. Depression as a risk factor for osteoporosis. Trends in Endocrinology & Metabolism. 2009;20(8):367-73.
- Hertel KL, Trahiotis MG. Exercise in the prevention and treatment of osteoporosis: the role of physical therapy and nursing. Nursing Clinics of North America. 2001 Sep 1;36(3):441-53.
- Sun S, Sun L, Kang Y, Tang L, Qin YX, Ta D. Therapeutic Effects of Low-Intensity Pulsed Ultrasound on Osteoporosis in Ovariectomized Rats: Intensity-Dependent Study. Ultrasound Med Biol. 2020 Jan;46(1):108-121. doi: 10.1016/j.ultrasmedbio.2019.08.025.
- Zhang W, Luo Y, Xu J, Guo C, Shi J, Li L, Sun X, Kong Q. The Possible Role of Electrical Stimulation in Osteoporosis: A Narrative Review. Medicina (Kaunas). 2023 Jan 8;59(1):121. doi: 10.3390/medicina59010121
- Chang KV, Hung CY, Chen WS, Lai MS, Chien KL, Han DS. Effectiveness of bisphosphonate analogues and functional electrical stimulation on attenuating postinjury osteoporosis in spinal cord injury patients-a systematic review and metaanalysis. PloS one. 2013;8(11): e81124.
- Wang J, Shang P. Static magnetic field: A potential tool of controlling stem cells fates for stem cell therapy in osteoporosis. Progress in Biophysics and Molecular Biology. 2022.
- Singh A, Varma AR, Varma A. Whole-Body Vibration Therapy as a Modality forTreatment of Senile and Postmenopausal Osteoporosis: A Review Article. Cureus. 2023;15(1).
- Cormick G, Belizán JM. Calcium intake and health. Nutrients. 2019 Jul 15;11(7):1606.
- De Martinis M, Allegra A, Sirufo MM, Tonacci A, Pioggia G, Raggiunti M, Ginaldi L, Gangemi S. Vitamin D deficiency, osteoporosis and effect on autoimmune diseases and hematopoiesis: a review. International journal of molecular sciences. 2021 Aug 17;22(16):8855.
- Uwitonze AM, Rahman S, Ojeh N, Grant WB, Kaur H, Haq A, Razzaque MS. Oral manifestations of magnesium and vitamin D inadequacy. The Journal of steroid biochemistry and molecular biology. 2020; 200:105636.
- Fusaro M, Cianciolo G, Brandi ML, Ferrari S, Nickolas TL, Tripepi G, Plebani M, Zaninotto M, Iervasi G, La Manna G, Gallieni M. Vitamin K and osteoporosis. Nutrients. 2020;12(12):3625.
- Li G, Zhang L, Wang D, AIQudsy L, Jiang JX, Xu H, Shang P. Muscle‐bone crosstalk and potential therapies for sarco‐osteoporosis. Journal of cellular biochemistry. 2019;120(9):14262-73.
- Hunter RW, Dhaun N, Bailey MA. The impact of excessive salt intake on human health. Nature Reviews Nephrology. 2022;18(5):321-35.
- Watanabe A, Miyajima K, Kemuriyama N, Uchiyama H, Anzai T, Iwata H, Anzai R, Nakae D. Impact of dietary calcium and phosphorus levels on an ovariectomized Sprague-Dawley rat model of osteoporosis. Fundamental Toxicological Sciences. 2019;6(7):253-8.
About the author
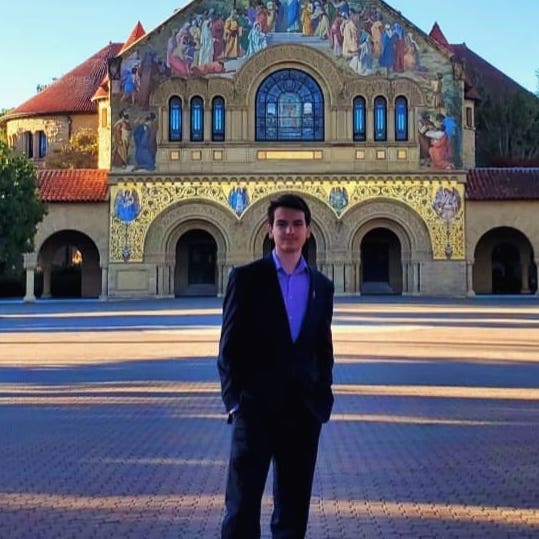
Milutin Todorovic
Milutin is a junior at Glenbrook South High School, located near Chicago. He is particularly interested in the intersection between econometrics and medical sciences. Outside of school, Milutin is involved in the Varsity Debate Team, Model United Nations, and being an Oralist for the Math Team; he enjoys playing Tennis in his free time, writing Columns for his school’s Newspaper (the Oracle), and being a club leader for the German National Honor Society.