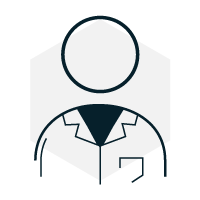
Author: Jiayi Huang
Mentor: Dr. Emma Sarro
The Pennington School
Abstract
Traumatic brain injury (TBI) and spinal cord injury cause many individuals to suffer each year. Current treatments for such neuronal injuries mainly rely on medication and surgeries. The recovery time usually takes years, depending on the individual. 3D cell cultivation, an emerging technique, may shorten the timespan of neuronal injury recovery by introducing lab-produced tissues into organisms to replace or supply the injured area. After reviewing recent research on 3D non-neuronal and neuronal cell culture, this review speaks to the promise of 3D cell culture in improving neuronal injury treatments.
Introduction
Traumatic brain injury (TBI) and spinal cord injury are neural injuries often resulting from falls, road accidents, or sport-related wounds. TBI is a sudden injury to the brain, usually occurring after a traumatic bump or jolt. It ranges from mild, moderate, to severe. While mild TBI, such as concussion, only causes temporary symptoms, including confusion and concentration difficulty, severe TBIs can cause coma, permanent brain damage, disability, and even death (Galgano et al, 2017).
In the Brain Injury Awareness Month in 2021, the BIAA reported that in the U.S., around 2,8 million people are subjected to TBI each year. Among the injuries, 50,000 are fatal, meaning that approximately 155 people die from TBI daily. In 2018, the global number of TBI sufferers was 69 million. Moreover, studies show a high mortality rate after severe TBI, ranging from 76% to 89%. In addition, according to the CDC, even after rehabilitation, about 50% survivors will undergo a decline in life or even die within 5 years after their injury (cdc.gov, 2022). https://www.cdc.gov/traumaticbraininjury/index.html )
Likewise, a traumatic spinal cord injury happens because of a sudden blow to the spine. It often leads to loss of strength and sensation below the injury site. A lower injury site may cause leg and lower body paralysis, while a higher injury site may affect most of the body and all limbs (clevelandclinic.org, 2020).
Approximately 17,810 cases of spinal cord injury emerge in the U.S. each year. According to the data from WHO in 2013, between 250,000 and 500,000 individuals suffer from SCI around the world annually (who.int). (https://www.who.int/news-room/fact-sheets/detail/spinal-cord-injury)
Both brain injury and spinal cord injuries lead to long term deficits in a similar way and under similar mechanisms. While they are similar in mechanism, they may lead to different kinds of sensory and motor deficits as well as behavioral and cognitive problems.
TBI treatment modalities vary based on the severity of the injury and range from daily medication to specified surgeries. Mild TBI is often cured from sufficient rest and sometimes
with assistance of pain-relieving medicines. Moderate to severe TBIs are treated with more complicated strategies, including emergency treatments, surgeries, and rehabilitation therapies. Emergency treatments ensure control of brain and blood pressure by using CSF drainage to lower ICP immediately by reducing intracranial volume. After the stabilization, severe patients often directly move into the operation room, relying on surgeries to reduce brain pressure by removing the swelling organs. A typical surgery is the evacuation of hematoma due to its compression of the brain. Rehabilitation of TBI includes physical therapy, speech therapy, and psychological care (nichd.nih.gov, 2020).
Similar to that of the TBI, treatments for SCI include medication while placing emphasis on surgery. Surgeries for SCI involve removing bone fragments and foreign objects that appear to be pressuring the spine (Spinalcord.com Team, 2020).
Since CNS neurons do not modulate growth-associated genes when reached their mature status, adult CNS neurons lose their ability to regenerate. Therefore, none of the methods mentioned above take into account how cells regrow. The current treatments focus on physical rebuild, which unfortunately makes full recovery after TBI and SCI take longer. While some patients may improve in six months, many people take one year to two or more years to show signs of recovery.
One avenue for treatment could come from stem cell research- specifically stem cell 3D culture. It has been tested in numerous types of tissues and cells and across animal models. Thus, 3D neuronal cultures may be a new avenue to research for treatment of the above injuries or disorders. This paper will explore the possibility and compare the current understanding with treatments now used for TBI’s or Spinal cord injury.
Methods
For this review, a literature research was carried out. Information was gathered primarily on databases PubMed, Google Scholar, and Science Direct using keywords include 3D cell culture, stem cells, neuronal cells, nonneuronal cells, organoids, traumatic brain injury, spinal cord injury, etc. The time parameter was limited to the past 10 years, mostly in the past 5 years, during which most advances in 3D cell culture was achieved.
To ensure the relativity of the information, only reviews that specified their 3D culture tools, process, origin of derived cell, and cultivation outcome were kept. These components provide a clear general picture of how the cultivation was performed, allowing this review to better summarize the development as well as deficiencies of 3D cell culture. All studies gathered had successfully produced stem cell organoids or even tissues using cell resources derived from either humans or mice.
Since this review focuses on neuronal stem cells, around half of the incorporated reviews are about neuronal cells. The other half are nonneuronal cells, ranging from endodermal liver, mesodermal intestine and bones, to ectodermal skin and retina. The broad non-neuronal cell research range served a pivotal role in paving the way for the more complex research on neuronal cells.
Results
Both 3D cultivation of neuronal and nonneuronal stem cells made progress, with nonneuronal cells better and more broadly researched. In the following paragraphs, summary of both cell type cultivation will be discussed. Non-neuronal cell research will be provided in the order of endodermal germ layer, mesodermal germ layer, and ectodermal germ layer. This is followed by summaries of neuronal cell researches, which range from neuronal stem cells, spheroids, to brain tissues.
Promising 3D methods
Endoderm/Mesoderm/Ectoderm
Endoderm: 3D cell culture across many cell types reached successes in creating organoids. To develop a liver transplantation therapy method free of the donor scarcity problem, researchers from Chinese PLA General hospital planted adipose-derived mesenchymal stem cells (ADSCs) and bone marrow-derived mesenchymal stem cells (BMSCs) on RSF scaffolds. Subsequently adding mesenchymal stem cells (MSC) on the scaffold complex, on which the MSCs differentiated to hepatocyte-like cells. Mice with injured liver function showed great therapeutic ability when injected with the MSCs–RSF matrices (Xu et al, 2017).
Mesoderm: Crypt- base columnar (CBC) cell, when received signal for growth, is able to generate a crypt-like ‘‘organoid’’ in 3D culture. A combination of CBC cells and Paneth cells better simulate the in vivo environment, allowing an intestinal organoid to form more efficiently (Sasai et al, 2013).
A more popular organoid formation in mesoderm would be bone marrow. To improve the efficiency of stem cell therapy for mandibular bone regeneration, researchs built matrigel-infiltrated Ti6Al4V porous scaffolds to culture bone marrow-derived mesenchymal stem cells (BMSCs). Mandibular-defected mice showed better new bone formation when treated with such scaffolds than mice with local BMSC injection or Matrigel treatment (Yu et al, 2018).
Articular cartilage has an insufficient self-healing capacity given its low cellularity and avascular features. After comparison in mice, researchers found that NF PLLA scaffold treated with Matrilin-3 (MATN3), a non-collagenous, cartilage-specific ECM protein enhances cartilage regeneration (Liu et al, 2018).
The ectodermal germ layer has progressed in 3D culture as well. Skin equivalents were built from keratinocytes in 3D culture, enabling skin pieces to be accessed cheaper and more reliable compared to using the traditional tissue engineering process (Szymański et al, 2020). In addition, retina organoids are constructed from human pluripotent stem cells (hPSCs). Generating retinal organoids from 16 hPSC lines provided broader regions of the retina to be assessed to avoid selection bias (Capowsk et ali, 2019).
Neuronal cell lines:
Besides 3D culture of non-neuronal stem cells, that of the neuronal stem cells also advanced. Utilizing computer-assisted laser-based fabrication techniques and iPSC-derived neuronal cells, two-photon polymerization (2PP-DLW) fabrication became a novel culture platform based on tubular microtowers (Turunen et al, 2017). Also using hPSC-derived neurons, along with an alternation of the hydrogel matrix to better resemble cells’ actual 3D microenvironment, researchers produced a 3D cell scaffold merely in a single process (Honkamäki et al, 2021).
Multiple studies focus on neuronal stem cells. A 3D murine brain tissue was generated from murine neural stem cells. Excitingly, the murine brain is responsive to external stimuli. This makes a convenient way of studying neuronal intercellular interactions (Caroll et al, 2021).
A number of tissues were successfully produced as well. To investigate if pro-neuronal cells can be created in vitro, researchers created a brain-like tissue located in the cortical spheroids using human iPSCs (Song et al, 2019).
Brain tissue, in particular cerebral organoids, was produced and was able to mimic the endogenous developmental program (Lancaster et al, 2014). Another self-organized polarized cerebellar structure was made through human embryonic stem cells (ESC) in order to understand how cells and neurons cooperate to form the cerebellar structure (Muguruma et al, 2015).
Furthermore, hPSC-derived axial stem cells were induced to become spinal cord neurons and skeletal muscle cells, which then self-organized and generated human neuromuscular organoids (NMOs). The NMO would then be used to study pathology and model neuromuscular diseases (Martins et al, 2020).
Potential pitfalls:
However, deficiencies remain in current 3D cultures. For instance, intestinal villus structures fail to emerge in the intestinal organoid, suggesting that extra support from nonepithelial components of the submucosa may be required.
While in vitro testings of neuronal stem cells seem prevalent in the review, that of the in vivo testings are rare. Most 3D neuronal cell cultivation reached the point of testing responses of the produced organoid or tissue in labs, but have not yet implanted the organoid into organisms. Because of this, we cannot certify whether the tissue is guaranteed to work in organisms.
Discussion
We showed the advancements of 3D cell culture in both non-neuronal stem cells and neuronal stem cells. Plentiful and diverse organoids were produced from different stem cell sources. Current stem cell-derived brain organoid enables testing for diseases and pathology, modeling neuronal diseases and their later events such as neuron maturation.
While conventional 2D cell culture allows scientists to study the cells in labs, 3D culture advances the application by replacing the flat surface culture environment with a three-dimensional space. The space better simulates the actual environment where cells reside inside organisms, allowing lab cells to become more physiologically relevant and predictive. 3D culture methods also induce higher degrees of complexity and retain homeostasis longer. Hence, 3D culture enables scientists to observe more closely the reaction and interaction of cells when being injured, and test more relevant cell responses to treatments.
However, given the complex nature of the neuron system, the amount of neuronal stem cell research is sparse and differs across injury types. In vivo tissue reaction toward the produced organoids has not yet occurred either. Therefore, future studies may focus on trying to implant the culture result into an organism to see actual treatment effects.
Conducting 3D cell culture opens the way for exploring more effective treatments. It enables a more comprehensive grasp of cell interactions, an understanding of cell reactions to treatments, and a faster and more convenient method of testing. 3D cell culture accelerates the pace and strengthens the potential for finding new treatments.
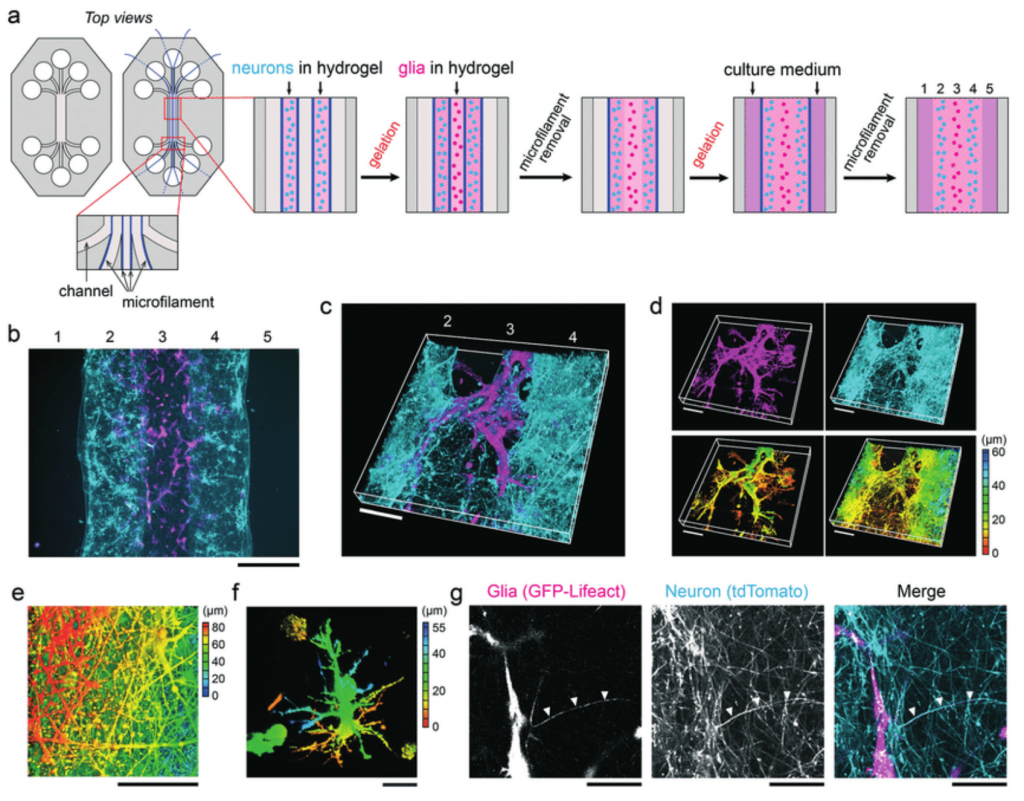
References
Capowski, E. E., Samimi, K., Mayerl, S. J., Phillips, M. J., Pinilla, I., Howden, S. E., Saha, J., Jansen, A. D., Edwards, K. L., Jager, L. D., Barlow, K., Valiauga, R., Erlichman, Z., Hagstrom, A., Sinha, D., Sluch, V. M., Chamling, X., Zack, D. J., Skala, M. C., & Gamm, D. M. (2019). Reproducibility and staging of 3D human retinal organoids across multiple pluripotent stem cell lines. Development (Cambridge, England), 146(1), dev171686. https://doi.org/10.1242/dev.171686
Carroll, J. A., Foliaki, S. T., & Haigh, C. L. (2021). A 3D cell culture approach for studying neuroinflammation. Journal of neuroscience methods, 358, 109201. https://doi.org/10.1016/j.jneumeth.2021.109201
Faustino, Martins, J. M., Fischer, C., Urzi, A., Vidal, R., Kunz, S., Ruffault, P. L., Kabuss, L., Hube, I., Gazzerro, E., Birchmeier, C., Spuler, S., Sauer, S., & Gouti, M. (2020). Self-Organizing 3D Human Trunk Neuromuscular Organoids. Cell stem cell, 26(2), 172–186.e6. https://doi.org/10.1016/j.stem.2019.12.007
Galgano, M., Toshkezi, G., Qiu, X., Russell, T., Chin, L., & Zhao, L. R. (2017). Traumatic Brain Injury: Current Treatment Strategies and Future Endeavors. Cell transplantation, 26(7), 1118–1130. https://doi.org/10.1177/0963689717714102
Honkamäki, L., Joki, T., Grigoryev, N. A., Levon, K., Ylä-Outinen, L., & Narkilahti, S. (2021). Novel method to produce a layered 3D scaffold for human pluripotent stem cell-derived neuronal cells. Journal of neuroscience methods, 350, 109043. https://doi.org/10.1016/j.jneumeth.2020.109043
Lancaster, M. A., & Knoblich, J. A. (2014). Generation of cerebral organoids from human pluripotent stem cells. Nature protocols, 9(10), 2329–2340. https://doi.org/10.1038/nprot.2014.158
Liu, Q., Jun, W., Chen, Y., Zhang, Z., Saunders, L., Schipani, E., Chen, Q., Ma, X. P. (2018) Suppressing mesenchymal stem cell hypertrophy and endochondral ossification in 3D cartilage regeneration with nanofibrous poly(l-lactic acid) scaffold and matrilin-3. Science Direct. Volume 76, 2018, Pages 29-38. https://doi.org/10.1016/j.actbio.2018.06.027
Muguruma, K., Nishiyama, A., Kawakami, H., Hashimoto, K., & Sasai, Y. (2015). Self-organization of polarized cerebellar tissue in 3D culture of human pluripotent stem cells. Cell reports, 10(4), 537–550. https://doi.org/10.1016/j.celrep.2014.12.051
Sasai, Y., (2013) Next-Generation Regenerative Medicine: Organogenesis from Stem Cells in 3D Culture. Cell Stem Cell Ya12. 520-530. http://dx.doi.org/10.1016/j.stem.2013.04.009
Song, L., Yuan, X., Jones, Z. et al. (2019). Assembly of Human Stem Cell-Derived Cortical Spheroids and Vascular Spheroids to Model 3-D Brain-like Tissues. Sci Rep 9, 5977. https://doi.org/10.1038/s41598-019-42439-9
Steppacher, I., Kaps, M., & Kissler, J. (2015). Against the odds: a case study of recovery from coma after devastating prognosis. Annals of clinical and translational neurology, 3(1), 61–65. https://doi.org/10.1002/acn3.269
Szymański, Ł., Jęderka, K., Cios, A., Ciepelak, M., Lewicka, A., Stankiewicz, W., & Lewicki, S. (2020). A Simple Method for the Production of Human Skin Equivalent in 3D, Multi-Cell Culture. International journal of molecular sciences, 21(13), 4644. https://doi.org/10.3390/ijms21134644
Turunen, S., Joki, T., Hiltunen, M., Ihalainen, T., Narkilahti, S., & Kellomäki, M. (2017). Direct Laser Writing of Tubular Microtowers for 3D Culture of Human Pluripotent Stem Cell-Derived Neuronal Cells. ACS Applied Materials & Interfaces 9 (31), 25717-25730 DOI: 10.1021/acsami.7b05536
Xu, L., Wang, X., Sui, X., et al. (man2017). Mesenchymal Stem Cell-Seeded Regenerated Silk Fibroin Complex Matrices for Liver Regeneration in an Animal Model of Acute Liver Failure. ACS Applied Materials & Interfaces 2017 9 (17), 14716-14723 https://doi.org/10.1021/acsami.7b02805
Yamada, A., Renault, R., Chikina, A., et al. (2016). Transient microfluidic compartmentalization using actionable microfilaments for biochemical assays, cell culture and organs-on-chip. ResearchGate, 2016,16, 4691–4701. DOI: 10.1039/C6LC01143H
Yu, L., Wu Y., Sui, X., Liu, J., et al. (2018). 3D Culture of Bone Marrow-Derived Mesenchymal Stem Cells (BMSCs) Could Improve Bone Regeneration in 3D-Printed Porous Ti6Al4V Scaffolds. Stem Cells International, vol. 2018, 13 pages. https://doi.org/10.1155/2018/2074021
Websites:
Centers for Disease Control and Prevention, National Center for Injury Prevention and Control, March 21, 2022 Traumatic Brain Injury and Concussion. https://www.cdc.gov/traumaticbraininjury/index.html
NICHD. Office of Communications. November 24, 2020. What are the treatments for traumatic brain injury (TBI)? https://www.nichd.nih.gov/health/topics/tbi/conditioninfo/treatment
World Health Organization. November, 2013. Spinal Cord Injury. https://www.who.int/news-room/fact-sheets/detail/spinal-cord-injury
National Spinal Cord Injury Statistical Center, Facts and Figures at a Glance. Birmingham, AL: University of Alabama at Birmingham, 2020. https://www.nscisc.uab.edu/Public/Facts%20and%20Figures%202020.pdf
Spinalcord.com Team. December 06, 2020. Everything You Need to Know about Spinal Cord Injury Surgery. https://www.spinalcord.com/blog/everything-you-need-to-know-about-spinal-cord-injury-surger y
Cleveland Clinic Organization. December 1, 2020. What is a spinal cord injury https://my.clevelandclinic.org/health/diseases/12098-spinal-cord-injury#:~:text=The%20spinal% 20cord%20relays%20messages,traumatic%20blow%20to%20the%20vertebrae.
About the author
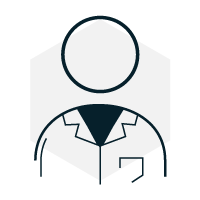
Jiayi Huang
Jiayi is a senior at The Pennington School. He is invested in cellular biology, with a particular interest in stem cells. In addition to participating in multiple biological programs and independent research in recent years, Jiayi became a medical lab and research assistant. Besides academics, Jiayi loves traveling and visual art. She held her first art exhibition in the Summer of 2022.