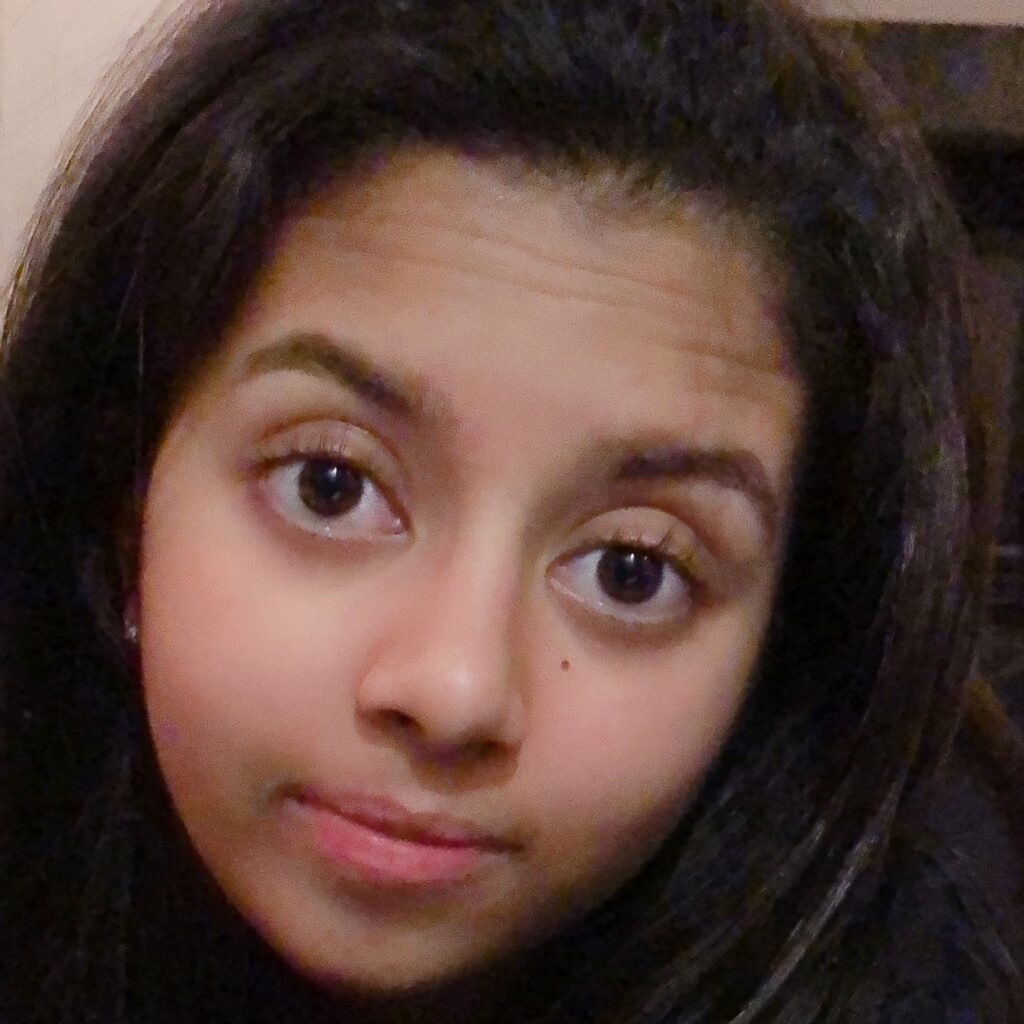
Author: Rishya Gutti
Mentor: Dr. Fabiola Munarin
Neuqua Valley High School
Abstract
Heart attacks, or Myocardial Infarctions (MI), lead to death of tissue due to lack of blood supply to the portion of the organ. Resulting scar tissue does not contract or function as well as healthy muscle tissue. On the other hand, stem cells have shown propensity to be guided into becoming specific cells that can be used to regenerate and repair diseased or damaged tissues in people. This paper will explore the latest research that supports using induced pluripotent stem cells for tissue reparation in cardiovascular disease.
Introduction
Over the past few decades, stem cell therapies have evolved considerably and one of their many potential applications could be to repair the scarring caused by myocardial infarctions. Myocardial Infarction (MI), which is a reduction or blockage of blood flow in the coronary arteries, commonly referred to as heart attack, is one of the leading causes of death in the United States with 805,000 people experiencing one every year (CDC). Unfortunately, out of those 805,000, 12% will die (CDC). Following MI, the inadequate blood flow to the infarcted tissue causes a severe reduction of oxygen and nutrients, leading to cardiomyocyte necrosis (reduced contractility), and therefore compromised heart function. MI does not traditionally have any treatment since once the tissue has necrotized, it can not regain its function. MI’s can only be managed with preventative measures taken to inhibit another incident. Medicines like aspirin and other anti-clotting drugs are used to keep clots from forming and causing another MI (CDC). ACE inhibitors reduce the strain on the heart by lowering blood pressure and this helps to not weaken the damaged tissues any further (NIH). Similarly, Betablockers also reduce the strain on the organ by blocking the release of stress hormones like noradrenaline and adrenaline to keep heart rate constant (NIH). All of these, however, only reduce the risk of a recurrence and do not regenerate the dead tissue, whereas a different form of therapy for the damaged tissue could bring about a brighter prospect. Stem cells can regenerate tissues suitable to one’s own body without having to use a transplant. This makes it less risky when it comes to a patient’s body rejecting the cells. Some varieties are also easily accessible, usable, and effective in their respective needs.
Out of the many varieties of stem cells, induced pluripotent stem cells, are some of the most promising to study. Induced pluripotent stem cells (iPS cells) are derived from somatic cells that are reprogrammed into iPS cells. These cells can then be made to differentiate into whatever tissue cell is needed (Shi et al, 2016). They are also important to observe because of their accessibility and high turnover rate (Krzysztof et al, 2018). In this review, we will focus on two types of stem cells: induced pluripotent stem cells, and their abilities in tissue regeneration in regards to therapies to treat the infarcted myocardium (Yoshida et al, 2017).
Induced Pluripotent Stem cells
Induced Pluripotent Stem Cells (iPSCs) are adult somatic cells that are reprogrammed into a pluripotent state. These cells are adults and unipotent, meaning they are capable of regenerating only their own specific tissue type (Tweedell, 2017). For example, an adult somatic cell in the skin could only generate skin cells. When these cells are reprogrammed into iPSCs, they become pluripotent, and are able to differentiate into any type of tissue with appropriate differentiation factors (Tweedell, 2017).
The use of iPSCs for regenerative medicine bears significant advantages. In fact, the somatic cells generally used for reprogramming are highly accessible and they are already part of the body of the person who needs them. Therefore, there is no risk of rejection when they are implanted for regenerating damaged tissues or organs (Arjmand et al, 2017). One further advantage is that they are not controversial like embryonic stem cells that are isolated from embryos while having similar properties. Generating the iPSCs is completed by taking any healthy adult somatic cells from the body and reverse engineering them into a pluripotent state where they can then differentiate into whatever cell type is needed. How this occurs is that first, the cells organize spatially and then divide into three areas. The middle section, differentiates into the middle portion of the three’s lineage and this activates certain genes.
Cardiovascular Regeneration
As mentioned above, one of the capabilities of iPSCs is tissue regeneration, which is paramount for cardiovascular tissue regeneration. The basic process for cardiovascular tissue engineering consists in isolating somatic cells of the patient or from healthy donors, which are then reprogrammed to iPSCs. Next, the obtained iPSCs are differentiated into the specific cell type that is needed (such as cardiomyocytes, cardiac fibroblasts, or endothelial cells). The differentiated cells must be cultured in the lab to grow, and during this process they can be stimulated with chemical or physical cues to mimic the mechanical properties of the beating heart. The last step is to inject or implant the cells into the patient.
Cardiovascular tissue engineering has shown promising results in vitro and in preclinical in vivo studies. Several groups have used small animal models, including mice and rats model of myocardial infarction to assess the ability of repairing the damaged heart tissue with iPSCs-derived cardiomyocytes. An example of implantation of cardiac engineered tissues in a small animal model is provided by (Tompkins et. al. 2018), that used 3D bioprinted iPSC-derived cardiomyocytes, fibroblasts and endothelial cells to produce 3D patches that were implanted in n=6 infarcted rats.
Additionally, vivo models further demonstrate that this path of study is incredibly promising. The work of Tompkins et. al. describes small animal models where iPSCs are implanted. This article demonstrates how this work is viable in live models as they tested various species of small animals to prove efficacy. Moreover, the same study considered large animal studies and deduced that they too have promising results. More specifically, in swine models, which are known to have extremely similar cardiac structure to that of humans, these studies further the thought that using iPSCs to repair tissues is a viable solution. Kawamura et al. placed a sheet of dermal fibroblast-derived hiPSC-CMs over the infarcted area in an ischemic swine model, which produced improved cardiac performance, angiogenesis (increased number of blood vessels in the infarct), and an attenuated LV remodeling 8-weeks post implantation.
While in the lab, stimuli of stretching and current are used to help the cells mature faster and grow more resilient. This is one place of research that is continuing to challenge researchers, since they do not have years to culture mature cells and there is risk with implanting immature cells regarding their ability to adapt to the heart’s environment. However, it can and has been done, as explained above, which has drawn tremendous attention to this field of pursuit. Moreover, cardiovascular regeneration is one of the newest technologies in repairing damaged tissues in the heart. This breakthrough has made it possible to just regrow healthy and functional tissue instead of needing a transplant since it is already known that once tissue is dead from a myocardial infarction, there is no way to salvage it. As the MI damages the tissue, it makes it impossible for the original tissue to be functional, so inputting fresh, cultivated tissues open up new possibilities in life for the patient after their MI episode.
Drug Screening
During the process of drug screening, various drugs are tested on the cardiac engineered tissues to gauge safety and efficacy of the tested molecules and drugs. One of the commonly tested side effects of newly developed drugs are for drug induced arrhythmias. By testing in-vitro with iPSCs outside of a patient’s body, it is not only more convenient to do so but also safer so as to not involve a live subject (Smith et. al. 2017). Various types of cell models are used, ranging from flat, 2D monolayers to more complex 3D tissues, organ-on-a-chip models show a wide range of functionality. Each of these model types show a range from the least to most complex levels of organization in order to understand how drugs can affect the cardiomyocytes on a basic to fully vascularized level (Smith et. al. 2017 Fig. 1). This is one place of development in the field of iPSCs that would be of great benefit to the scientific community and to the general population as well. If drugs can be screened and tested within a lab without having to use in vivo models until much later in the process, it can be much more ethical and more varieties of medicines that may or may not be viewed as viable could potentially be trialed in this way due to the reduced ethics concerns.
One example of a clinical trial is in the research of Blinova et. al. which shows a personalized drug screen model that highlights how iPSCs derived from 22 healthy subjects can be grown and tested within a dish. Safety and efficacy of two drugs, dofetilide and moxifloxacin (hERG‐blocking and QT prolonging), were tested on iPSCs isolated from the peripheral blood mononuclear cells and differentiated in cardiomyocytes. There were no drug induced arrhythmia-like events observed at the studied drug concentration rate. In vivo model of testing that highlights how tissue can be grown and tested within a dish. In this trial, the researchers tested and analyzed for arrhythmias in the iPSCs. This demonstrates how various environments of the heart can be simulated in the lab and that it is necessary to do so (Blinova et. al. 2019)
Various different types of trials can be used to screen for arrhythmias in a drug screening. One method researchers historically and commonly use is the analysis of hERG channel response which is the standard procedure for in vitro preclinical trials of drug screening. While this is a method commonly used, it is not as accurate as could be desired since false positive results are frequent occurrences (Smith et. al. 2017). This is why iPSC-CMs are making headway in the field of drug screening since they offer a more accurate option. There are various tests researchers can run with in vitro models of iPSCs to represent the function of the heart more fully and effectively. Out of the multitude of options researchers now have with iPSC-CMs as an option, an example presented in the above research is that researchers measure cell contraction to observe the cardiomyocytes’ contractile function (Smith et. al. 2017).
Conclusion
After an episode of myocardial infarction, heart tissue is damaged irreversibly and the prognosis only entails either drug therapeutics or organ transplant. Cardiovascular regeneration is one of the newest technologies in regards to repairing damaged tissues in the heart. With reprogrammed iPSCs , the patient is able to have their healthy cells cultured in a lab and remediate the scarred tissue resulting from an MI episode. Furthermore, progress has also been made in labs to accommodate the new research and to screen drugs to ensure their safety with the cultured tissues when implanted in a person. All together, these breakthroughs have made it possible to regrow healthy and functioning tissue and using iPSCs could make this possibility a reality.
References
Arjmand B, Goodarzi P, Mohamadi-Jahani F, Falahzadeh K, Larijani B. Personalized Regenerative Medicine. Acta Med Iran. 2017 Mar;55(3):144-149.
Blinova K, Schocken D, Patel D, Daluwatte C, Vicente J, Wu JC, Strauss DG. Clinical Trial in a Dish: Personalized Stem Cell-Derived Cardiomyocyte Assay Compared With Clinical Trial Results for Two QT-Prolonging Drugs. Clin Transl Sci. 2019 Nov;12(6):687-697.
CDC, Heart Disease in the United States, 2020 Sept.
Csöbönyeiová M, Polák Š, Danišovič L. Perspectives of induced pluripotent stem cells for cardiovascular system regeneration. Exp Biol Med (Maywood). 2015 May;240(5):549-56.
Hoang P, Wang J, Conklin BR, Healy KE, Ma Z. Generation of spatial-patterned early-developing cardiac organoids using human pluripotent stem cells. Nat Protoc. 2018 Apr;13(4):723-737.
Justin Liu, Kathleen Miller, Xuanyi Ma, Sukriti Dewan, Natalie Lawrence, Grace Whang, Peter Chung, Andrew D. McCulloch, Shaochen Chen,Direct 3D bioprinting of cardiac micro-tissues mimicking native Biomaterials, Volume 256, 2020, 120204, ISSN 0142-9612myocardium,
Kawamura M, Miyagawa S, Miki K, Saito A, Fukushima S, Higuchi T, Kawamura T, Kuratani T, Daimon T, Shimizu T, Okano T, Sawa Y. Feasibility, safety, and therapeutic efficacy of human induced pluripotent stem cell-derived cardiomyocyte sheets in a porcine ischemic cardiomyopathy model. Circulation. 2012 Sep 11;126(11 Suppl 1):S29-37.
Madigan M, Atoui R. Therapeutic Use of Stem Cells for Myocardial Infarction. Bioengineering (Basel). 2018 Apr 6;5(2):28
Müller P, Lemcke H, David R. Stem Cell Therapy in Heart Diseases – Cell Types, Mechanisms andImprovement Strategies. Cell Physiol Biochem. 2018;48(6):2607-2655.
NIH, What is a Heart Attack?, 2018 June.
Polonchuk L, Chabria M, Badi L, Hoflack JC, Figtree G, Davies MJ, Gentile C. Cardiac spheroids as promising in vitro models to study the human heart microenvironment. Sci Rep. 2017 Aug 1;7(1):7005.
Rikhtegar R, Pezeshkian M, Dolati S, Safaie N, Afrasiabi Rad A, Mahdipour M, Nouri M, Jodati AR, Yousefi M. Stem cells as therapy for heart disease: iPSCs, ESCs, CSCs, and skeletal myoblasts. Biomed Pharmacother. 2019 Jan;109:304-313.
Shi Y, Inoue H, Wu JC, Yamanaka S. Induced pluripotent stem cell technology: a decade of progress. Nat Rev Drug Discov. 2017 Feb;16(2):115-130.
Smith AS, Macadangdang J, Leung W, Laflamme MA, Kim DH. Human iPSC-derived cardiomyocytes and tissue engineering strategies for disease modeling and drug screening. Biotechnol Adv. 2017 Jan-Feb;35(1):77-94.
Tompkins BA, Balkan W, Winkler J, Gyöngyösi M, Goliasch G, Fernández-Avilés F, Hare JM. Preclinical Studies of Stem Cell Therapy for Heart Disease. Circ Res. 2018 Mar 30;122(7):1006-1020.
Tweedell KS. The Adaptability of Somatic Stem Cells: A Review. J Stem Cells Regen Med. 2017 May 30;13(1):3-13
Ye L, Swingen C, Zhang J. Induced pluripotent stem cells and their potential for basic and clinical sciences. Curr Cardiol Rev. 2013 Feb 1;9(1):63-72.
Yoshida Y, Yamanaka S. Induced Pluripotent Stem Cells 10 Years Later: For Cardiac Applications. Circ Res. 2017 Jun 9;120(12):1958-1968.
Zhao Y, Rafatian N, Wang EY, Wu Q, Lai BFL, Lu RX, Savoji H, Radisic M. Towards chamber specific heart-on-a-chip for drug testing applications. Adv Drug Deliv Rev. 2020;165-166:60-76.
About the author
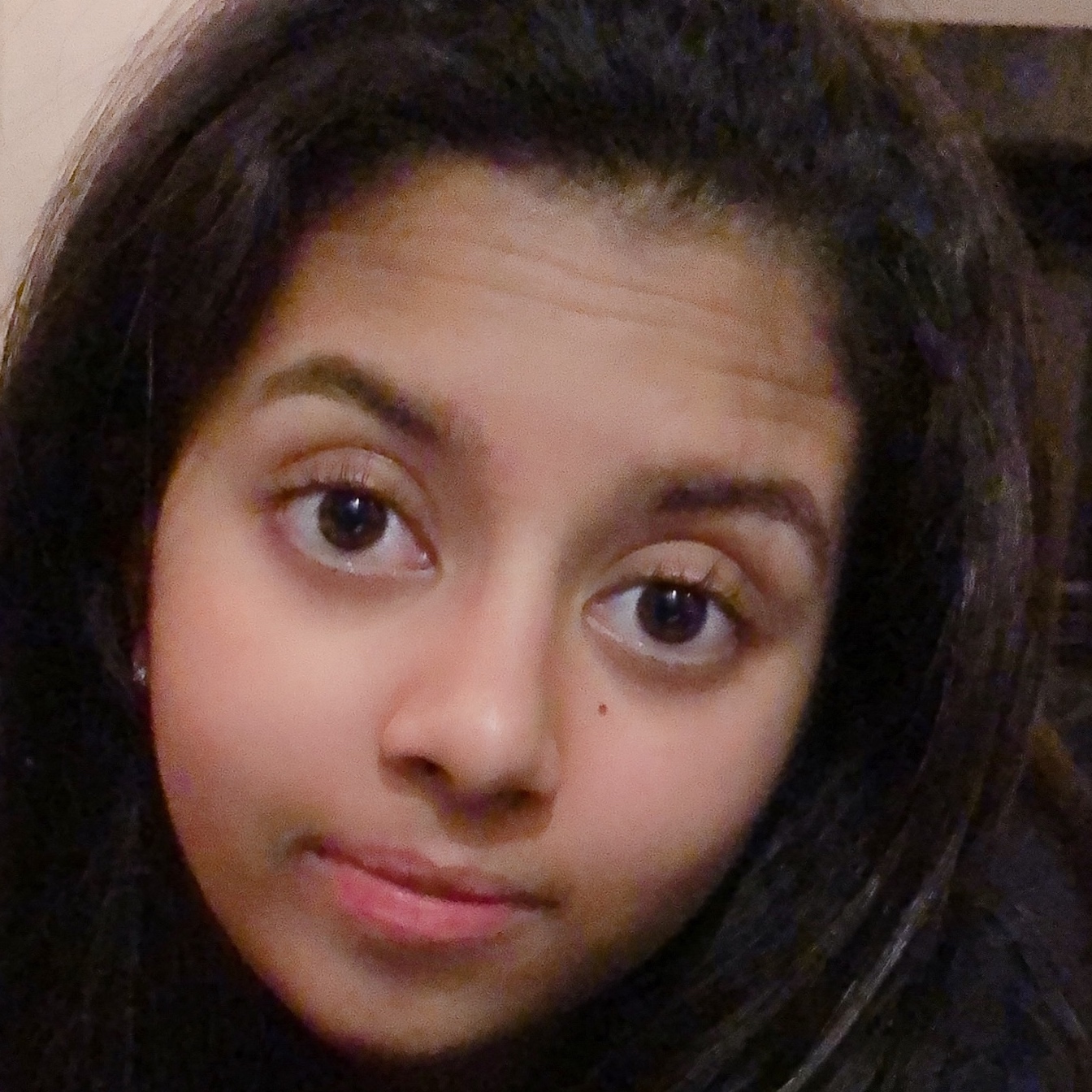
Rishya Gutti
Rishya is a junior at Neuqua Valley High School. She is interested in biological sciences and is an aspiring medical student. Research programs like RISE (Research, Inquiry Skills & Experimentation) have equipped Rishya with necessary skills to conduct independent research. She is a third degree black belt in Taekwondo and has won several national titles in her age group. Rishya enjoys volunteering her time to teach mathematics to younger students and to promote mental health awareness through a non-profit organization. In her free time, you will find her reading, working out, or watching her favorite tv shows.