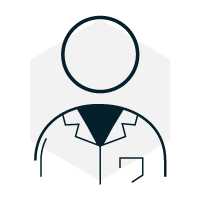
Author: Hao Shen
Mentor: Anthony Dichiara
Shanghai YK Pao School
Abstract
Perfluoroalkyl and poly-fluoroalkyl substances (PFAS), also known as “forever chemicals,” are widely put into application in agricultural and industrial fields. However, PFAS compounds have adverse health effects on the environment and the human body due to their permanent characteristics. In the past two decades, many countries have been raising concerns regarding to the impact of PFAS and gradually phasing out the use of PFAS but have found the remediation process particularly costly and difficult to operate. This report reviews the current removal of PFAS through continuous flow sorption systems and provides a review of the recent scientific literature on the aqueous-phase adsorption of PFAS in batch and fixed bed systems using green sorbent materials such as agricultural wastes and polysaccharide-based materials.
Keywords: PFAS, adsorption, water treatment, continuous flow system
Introduction
Perfluoroalkyl and poly-fluoroalkyl substances, also known as PFAS, are a group of synthetic chemicals characterized by aliphatic carbon backbones that are partially bonded with fluorine (poly-fluoroalkyl) or fully fluorinated (per-fluoroalkyl). Since fluorine is the most electronegative element among the halogens, the bond between carbon and fluorine in the PFAS compound results in strong hydrophobicity, and chemical and thermal stability, making them extremely resilient to oxidation and degradation from heat, oil, and water. Due to these reasons, PFAS is also known as the ‘forever chemical’ and remains in the environment for significantly long periods of time. Such properties have provided an extensive application in industry and consumer products worldwide since the 1940s, mainly for protective coatings, waterproof fabrics, and firefighting foam; and can be found in a majority of daily products such as paints, shampoo, non-stick cookware, fast food packaging, stain resistance products, and pesticides. Throughout thousands of artificially created PFAS, perfluoro-octanoic acid (PFOA) and perfluoro-octane sulfonic acid (PFOS) are the most commonly studied. (Glüge, 2020)
Over the past two decades, most manufacturers in Canada and the United States have gradually phased out their use of PFAS, due to their permanent characteristics, they are still having adverse health effects on the environment and human bodies. (Singh, 2023) Institutions such United States Environmental Protection Agency (EPA) have proposed an action plan outlining the specific steps that the agency and stakeholders need to take to protect public health. (2023) In the action plan, drinking water is marked as a priority issue, with a focus on promoting the Maximum Pollutant Level (MCL) process for perfluoro-octanoic acid (PFOA) and perfluoro- octane sulfonic acid (PFOS). The institution is also collecting and evaluating information to determine whether regulation is suitable and viable for a wider range of PFAS categories. The main ways of people’s exposure to PFAS include drinking contaminated water from public and private water systems, consuming fish or food with a high level of PFAS, using food packaged in material made with PFAS, employment in a workplace that involves PFAS use, and household consumer products such as ski wax, nonstick cookware, and water repellant fabric clothing. As PFAS accumulates over time in the environment and bodies of humans and animals, PFAS’s toxicity is investigated by many animal studies, suggesting prominent environmental impact and adverse health issues, including major developmental impact on fetus during pregnancy, causing infant low birth weight, puberty changes, bone variations; testicles and kidneys cancer; and thyroid effects related to developmental outcomes. According to a European biomonitoring study, French and Sweden’s teenagers had the highest level of PFAS, with 11.26 and 12.31 micrograms per liter. (2022)
“The fact that these levels of PFAS can be found in our growing population is infuriating. Misleading industrial actors and lagging legislation have allowed these chemicals to poison too many generations already. It’s time to put an end to this madness once and for all,” suggested Dr. Jonatan Kleimark, ChemSec’s Senior Chemicals and Business Advisor. There are various existing and potential remediation solutions, along with national efforts to reduce PFAS risks to the public and move to less bio-accumulative materials.
Although many countries are phasing out the use of it, PFAS-contaminated surface water and groundwater have been increasingly detected among the ecological systems. The unique chemical properties of PFAS make it very effective in applications, but it is also particularly difficult to remediate. Therefore, this report will summarize and evaluate different remediation solutions, followed by a reasoned recommendation. There are currently various technologies available for removing PFAS from aqueous media, mainly led by two processes: degradation and separation. Degradation refers to the biotic or abiotic depolymerization of polymers into monomers (complete depolymerization) or oligomers and other chemicals (partial depolymerization), typically requiring a catalyst. Some of the degradation methods, including sonolysis, plasma treatment, electrochemical oxidation, and reduction, have been proven to be effective at the laboratory scale. However, the lengthy treatment time and costly energy requirements have made many regions unaffordable to execute on a large ecological scale. Separation, on the other hand, consists of membrane filtration and sorption which comprise adsorption and absorption. The size exclusion membrane filtration method is limited by the molecular size of PFAS compounds, as separation only occurs when the PFAS molecules are larger than the pores of the semi-permeable membrane. However, the separation process also presents challenges in removing target chemicals such as PFAS and other compounds that can be preserved in aqueous solutions. (Militao, 2021) Adsorption is an effective treatment technique due to its simple operation, high efficiency, and ability to remove toxic contaminants with exceedingly low concentrations. This method refers to the adhesion of molecules transferred from a fluid bulk (adsorbate) to a solid surface (adsorbent). (Lei, 2023)
Therefore, this report aims to provide an overview of studies on the removal of PFAS in continuous flow systems, compare renewable adsorbents in batch studies, and identify potential candidates for future continuous flow sorption research. Nevertheless, the removal of PFAS has been underestimated since there are many other studies on PFAS besides the ones shown in the figure that are not covered in this research, including key terms such as sorption and cleaning.
Results and discussions
General information and definitions
Currently, most published academic research is focused and limited to PFAS removal through batch processing systems that are conducted using single solute adsorption in pure water, which is not representative of real-world wastewater effluents. However, the results are usually overestimated due to unrealistic conditions. In most batch systems, the adsorbent experiment was conducted at a high PFAS concentration which promotes mass transfer for faster sorption kinetics but is not considered representative of the concentration level in nature. The regeneration and reuse or recycling of spent adsorbents remains a great challenge. Therefore, studies conducted through continuous flow processes are essential for predicting the effectiveness and practicality of adsorbents in real-world applications.
Empty bed contact time (EBCT) is considered to be a fundamental parameter in evaluating an fixed bed adsorption system, as studies have shown that it affects the removal of PFAS to varying degrees. It is a measure of time when the water to be treated flows into contact with the treatment medium in the container. (Pedia, 2017) And the level of breakthrough refers to the amount of pollutants filtered out from the contaminated water compared to the pollutant in pre- treatment water.
Review of previous studies
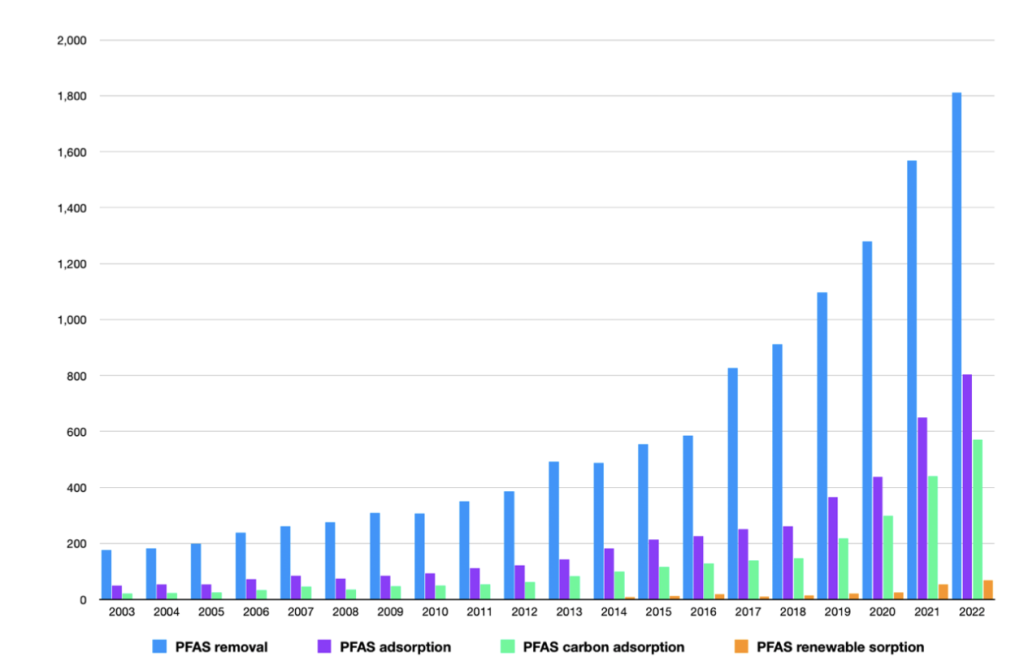
As shown in Figure 1, the number of peer-reviewed articles on PFAS removal has been continuously increasing over the past two decades, with an approximate increase of 94% between 2003 and 2022. Among all types of treatments, PFAS adsorption accounts for more than one-third of the total literature, indicating its effectiveness and recognition of the method in scientific research. More specifically, over 70% of them are investigation of carbon-based adsorbents, while in recent years, renewable materials that have just been put into research occupy for a minority among them, accounting for only 8% of the total. Among many sorptive treatments, granular activated carbon (GAC) and advanced ion exchange (AIX) are considered currently the most feasible solutions to carry out at a large scale. (Dixit, 2021)
According to the investigation that Murray, Marshall, Liu, and Vatankhah conducted, the two most studied adsorption treatments, granular activated carbon (GAC) and ion exchange resin (IX), were tested and directly compared in a continuous flow system set up by a segmented column system. (Murray, 2021) The experiment tested columns with different empty bed contact times and evaluated the optimal EBCT for GAC and IX treatment by obtaining potential mass transfer zones (MTZ) of PFAS. EBCT and MTZ have a high impact on the system’s operation costs and adsorption capacity; The cost of long EBCT is often excessively high, while the adsorbent utilization rate of short EBCT is insignificant. Therefore, by generating data on treatability through continuous flow columns, the adsorption capacity of GAC and IX for long- chain and short-chain PFAS could be calculated, to improve the system longevity, achieving an adsorption effect while minimizing operating costs.
The bench scale column system (Fig. 2) is comprised of six columns, divided into three segments with distinct sampling ports. During the operation, a constant flow rate through each column is generated by a constant head reservoir, which is sequentially connected to each column controlled by a drying medium. To test the impact of EBCT, the time was controlled at 3 minutes per GAC column and 1 minute per IX column.
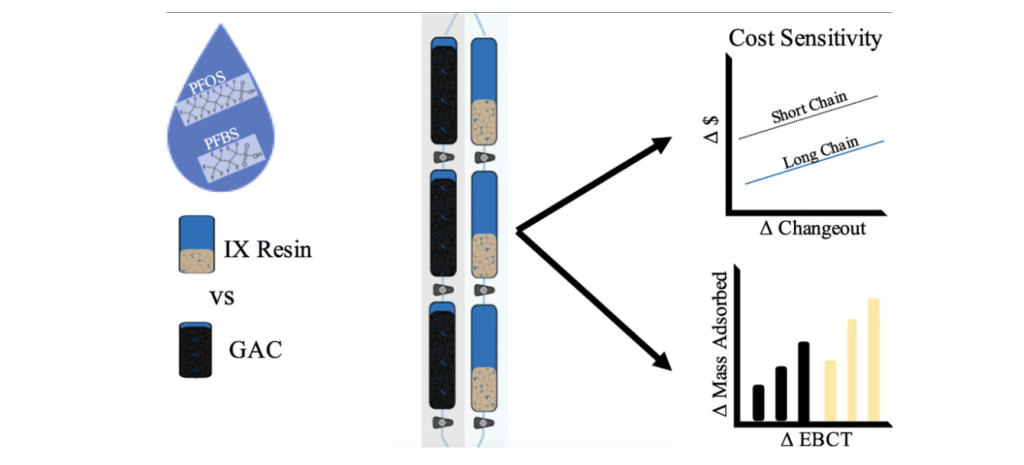
Note. Schematic of the lab scale column system. From
Murray, C. C., Marshall, R. E., Liu, C. J., Vatankhah, H., & Bellona, C. L. (2021). PFAS treatment with granular activated carbon and ion exchange resin: Comparing chain length, empty bed contact time, and cost. Journal of Water Process Engineering, 44, 102342. https://doi.org/10.1016/j.jwpe.2021.102342
The results of this study indicate that:
- In removing long-chain PFAAs, the effectiveness of GAC and IX depends on chain length and their functional group. According to its adsorption capacity and a further level of breakthrough, IX completely outperformed GAC treatment, most significantly in the removal of PFHxS and PFOS compounds. The minimum contact time required for effective long chain PFAS adsorption for GAC is 3 times that of IX.
- The adsorption capacity of GAC and IX is significantly lower for short-chain PFAAs, therefore, maintaining a low-level breakthrough of short-chain may be challenging. While the overall treatment performance of IX is inconspicuous, GAC treatment presented a prominent declining capacity in short-chain PFCAs.
- Compared with the long-chain PFAA of GAC and IX, MTZ has demonstrated that the treatment of short-chain PFAA requires longer EBCT. Therefore, it is necessary to handle short-chain PFAA with longer adsorption time and higher operating costs.
- IX adsorption treatment is more cost-effective than GAC while meeting both goals of removal of long and short-chain PFASs.
In summary, although granular activated carbon and ion exchange resin are considered the most viable treatment technologies for PFAS removal in current research when applied in full-scale continuous flow systems, they still have significant drawbacks: their high cost and its poor regeneration capability restrict their use at larger scale. Continuous efforts have been made to find more efficient and environmentally friendly sorbents; recently, the development of low-cost adsorbent alternatives derived from natural materials, industrial wastes, and agricultural byproducts has become crucial.
Literature comparison
Green adsorbents refer to those alternative materials to remove PFAS that are easy to produce, affordable, and environmentally friendly. At present, there is no clear direction or publications in the scientific field for “more environmentally friendly adsorbents”; therefore, this report aims to provide an overview of potential substitutes for traditional activated carbon and identify several possible candidates for future continuous flow studies that would be applied to a full-scale water treatment system. The existing greener adsorbents for PFAS remediation could be categorized into three types: activated carbon and biochar from agro-organic wastes, polysaccharide-based material, and animated and household or industrial wastes-based adsorbents. (Militao, 2021) Most of the raw materials are physically or chemically modified to maximize their adsorption capacity and selectivity. Agricultural wastes, such as agro-organic wastes, are widely used in the production of activated carbon; thus, these materials could also be used as reagents for adsorbing organic pollutants in water removal systems. Two of the mainly used adsorbents are grape leaf litter and bamboo AC. Polysaccharide-based material includes cross-linked chitosan beads and cellulose-based adsorbents such as PEI-f-cellulose. Due to its unique physical and chemical properties, such as the diversity of reaction groups, low cost, and availability, it has shown potential applications in water treatment. These natural polysaccharides come from renewable and biodegradable resources, such as chitin, cellulose, and starch. Although adsorbents based on animated household and industrial wastes are not extracted from natural materials, they are still advantageous when applied to the environment.
According to Table 1 shown in the Appendix, the adsorption kinetics and capacity for each green adsorbent are tested and compared. Among the different green adsorbents reviewed in this article, bamboo AC, graded microporous biochar (HMB), and cross-linked chitosan beads showed the highest adsorption capacity for PFAS, especially for long-chain PFAS, which have the potential to be applied in the commercial scale. More surprisingly, some of these green adsorbents even have a higher adsorption capacity for PFAS than many commercial GAC products that had been applied on a large scale. However, adsorbents such as cross-linked chitosan beads have presented a long equilibrium time, which would be a potential challenge in large-scale applications. Nevertheless, some of the statistics are underestimated and cannot be directly compared due to the difference in solution pH and the initial PFAS concentration. The research also indicates that electrostatic attractions and hydrophobic interactions have become critical mechanisms for PFAS removal.
Conclusion
Among the adsorbents investigated in the continuous flow system, ion exchange resin adsorption has exhibited potential over adsorption by the granular activated carbon both for the removal of long and short-chain PFASs. On the other hand, with the development of new renewable carbonaceous sorbents, new opportunities have also emerged in green adsorbents such as bamboo AC, graded microporous biochar (HMB), and cross-linked chitosan beads. Therefore, more research is needed to examine the potential of these renewable adsorbents in continuous flow processes, associating with more research investigating the regeneration of waste adsorbents that desorb PFAS from the sorbent surface and reuse it. At present, in order to mitigate the environmental impact of the regeneration process, thermal, microwave, and microbial methods have been considered in large-scale applications. (Gagliano, 2020)
References
Deng, S., Nie, Y., Du, Z., Huang, Q., Meng, P., Wang, B., Huang, J., & Yu, G. (2015). Enhanced adsorption of perfluorooctane sulfonate and perfluorooctanoate by bamboo-derived granular activated carbon. Journal of Hazardous Materials, 282, 150-157. https://doi.org/10.1016/j.jhazmat.2014.03.045
Deng, S., Niu, L., Bei, Y., Wang, B., Huang, J., & Yu, G. (2013). Adsorption of perfluorinated compounds on aminated rice husk prepared by atom transfer radical polymerization. Chemosphere, 91(2), 124-130. https://doi.org/10.1016/j.chemosphere.2012.11.015
Deng, S., Zheng, Y., Xu, F., Wang, B., Huang, J., & Yu, G. (2012). Highly efficient sorption of perfluorooctane sulfonate and perfluorooctanoate on a quaternized cotton prepared by atom transfer radical polymerization. Chemical Engineering Journal, 193-194, 154-160. https://doi.org/10.1016/j.cej.2012.04.005
Dixit, F., Dutta, R., Barbeau, B., Berube, P., & Mohseni, M. (2021). PFAS removal by ion exchange resins: A review. Chemosphere, 272, 129777. https://doi.org/10.1016/j.chemosphere.2021.129777
European teenagers are high – on PFAS. (2022, June 30). Retrieved September 19, 2023, from https://chemsec.org/european-teenagers-are-high-on-pfas/
Gagliano, E., Sgroi, M., Falciglia, P. P., Vagliasindi, F. G., & Roccaro, P. (2020). Removal of poly- and perfluoroalkyl substances (PFAS) from water by adsorption: Role of PFAS chain length, effect of organic matter and challenges in adsorbent regeneration. Water Research, 171, 115381. https://doi.org/10.1016/j.watres.2019.115381
Glüge, J., Scheringer, M., Cousins, I. T., DeWitt, J. C., Goldenman, G., Herzke, D., Lohmann, R., Ng, C. A., Trier, X., & Wang, Z. (2020). An overview of the uses of per- and-polyfluoroalkyl substances (PFAS). Environmental Science: Processes & Impacts, 22(12), 2345-2373. https://doi.org/10.1039/d0em00291g
Key EPA Actions to Address PFAS. (2023, April 21). Retrieved September 19, 2023, from https://www.epa.gov/pfas/key-epa-actions-address-pfas
Lei, X., Lian, Q., Zhang, X., Karsili, T. K., Holmes, W., Chen, Y., Zappi, M. E., & Gang, D. D. (2023). A review of PFAS adsorption from aqueous solutions: Current approaches, engineering applications, challenges, and opportunities. Environmental Pollution, 321, 121138. https://doi.org/10.1016/j.envpol.2023.121138
Militao, I. M., Roddick, F. A., Bergamasco, R., & Fan, L. (2021). Removing PFAS from aquatic systems using natural and renewable material-based adsorbents: A review. Journal of Environmental Chemical Engineering, 9(4), 105271. https://doi.org/10.1016/j.jece.2021.105271
Murray, C. C., Marshall, R. E., Liu, C. J., Vatankhah, H., & Bellona, C. L. (2021). PFAS treatment with granular activated carbon and ion exchange resin: Comparing chain length, empty bed contact time, and cost. Journal of Water Process Engineering, 44, 102342. https://doi.org/10.1016/j.jwpe.2021.102342
Pedia, M. (2017, September 20). Product Activated Carbon. Retrieved September 19, 2023, from https://www.urbansaqua.com/wp-content/uploads/2018/04/Mike-o- Pedia_Carbon_EBCT.pdf
Singh, I. (2023). Industry knew about risks of PFAS ‘forever chemicals’ for decades before push to restrict them, study says Social Sharing. CBC News. https://www.cbc.ca/news/science/pfas-3m-dupont-study-1.6862883
Zhang, Q., Deng, S., Yu, G., & Huang, J. (2011). Removal of perfluorooctane sulfonate from aqueous solution by crosslinked chitosan beads: Sorption kinetics and uptake mechanism. Bioresource Technology, 102(3), 2265-2271. https://doi.org/10.1016/j.biortech.2010.10.040
Zhou, Y., Xu, M., Huang, D., Xu, L., Yu, M., Zhu, Y., & Niu, J. (2021). Modulating hierarchically microporous biochar via molten alkali treatment for efficient adsorption removal of perfluorinated carboxylic acids from wastewater. Science of the Total Environment, 757, 143719. https://doi.org/10.1016/j.scitotenv.2020.143719
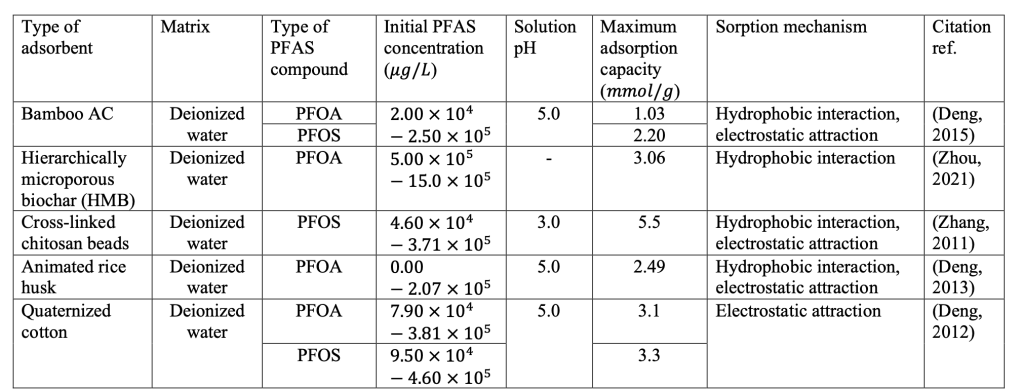
About the author
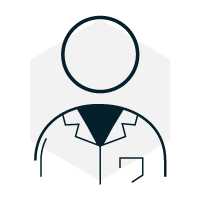
Hao Shen
Hao is a senior high school student from Shanghai YK Pao school. She is also a passionate and dedicated young environmental activist who is interested in environmental engineering.