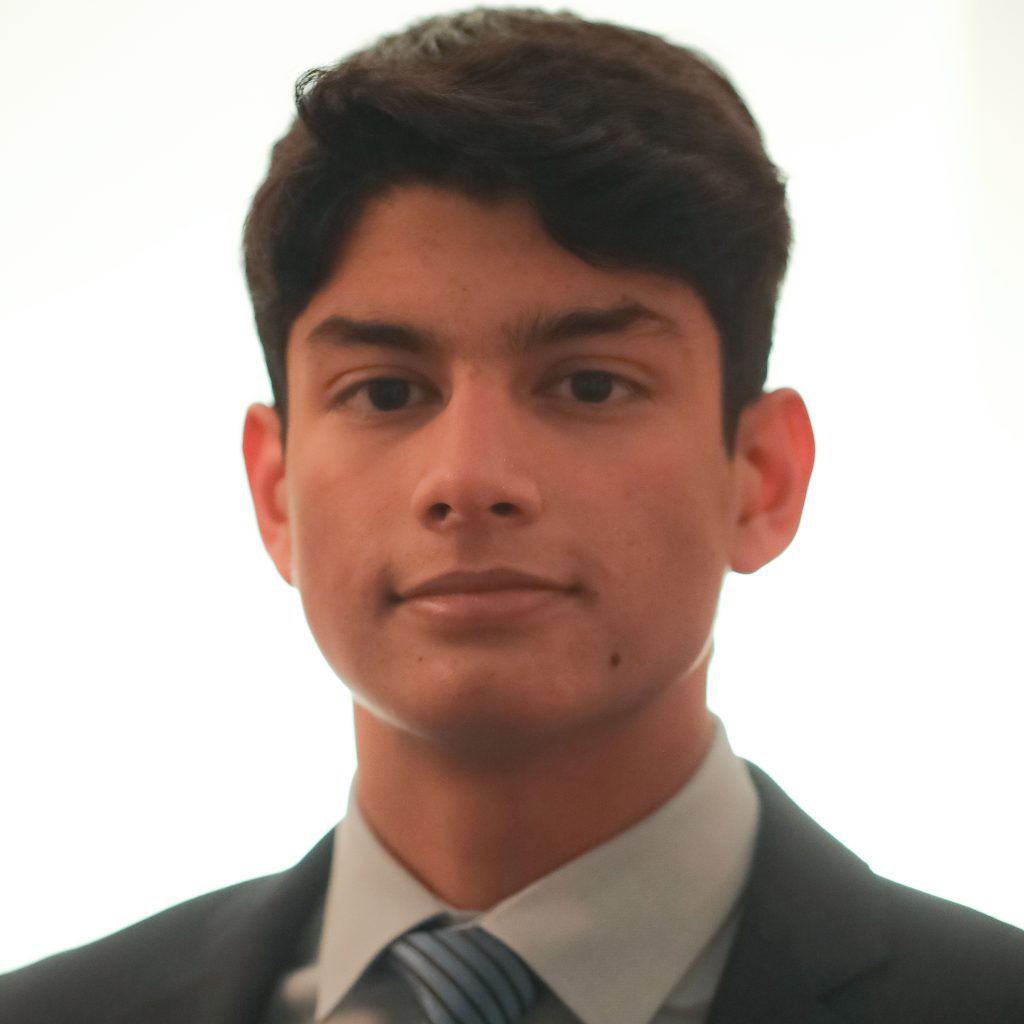
Author: Aniket Martins
Mentor: Dr. Ella Atkins
Fairfield Ludlowe High School
Introduction
Deep space missions have become the interest of space agencies over the past 50 years after humans successfully reached space and our moon. The technology required to explore the solar system and the universe outside our solar system has grown from simple rockets and capsules designed to bring people to the moon to impressive orbiters and satellites equipped with the latest in research technology to expand humanity’s understanding of our solar system and the universe that surrounds us.
This report will present the stories, physics, and science missions of four historic NASA deep space missions: Voyager, Pioneer (10 and 11), Galileo, and Cassini. These missions are of particular interest to me due to their role in boosting human understanding of various planets and the outer edges of the solar system. Voyager used gravity assist and a rare alignment of planets to research Jupiter, Saturn, Uranus, and Neptune. The Pioneer missions both flew by Jupiter and Pioneer 11 also conducted a detailed exploration of Saturn. Galileo sent a probe into Jupiter’s atmosphere and a spacecraft entered the Jovian orbit. Cassini used a probe and spacecraft to learn about Saturn, its rings, and its satellites as well. Combined these missions altered humanity’s concepts of space by confirming existing theories and presenting information that lead to new questions. This paper will analyze the science objectives of each mission, their orbits and launch vehicles, and other particularly interesting information. To conclude, the facts of previous missions will be used to propose ideas for new missions and offer commentary on established plans for future missions as well.
Outline of Missions
Voyager
The Voyager missions were launched in the 1970s to explore deep space beyond what humans had conceived before. Intended to flyby various bodies within the solar system and then continue gathering data outside of our solar system, the Voyager missions have been instrumental in learning about planets within our solar system and about the energy and space outside the solar system as well [1].
Science of the Mission
The Cosmic Ray Subsystem (CRS) looks for energetic particles in plasma and has high sensitivity. Such particles can be found in intense ration fields like the area around Jupiter, or from other stars. The CRS aimed to gather data to provide information on the content of energy and dynamics of cosmic rays across the galaxy in an attempt to better understand ray sources from across the galaxy, even beyond our star [2]. Highly energetic particles can be detected from fields around large planets such as Jupiter. Stars also emit high energy particles which the CRS system tracks. Since Voyager is now beyond our solar system in deep space, these readings provide scientists with important information about the universe that surrounds us.
Voyager also had a three-part Infrared Interferometer Spectrometer (IRIS) which acted as a thermometer, detecting temperature emissions from space bodies, a sensor for different elements and compounds near atmospheres of space bodies, and a radiometer to measure sunlight reflected at ultraviolet, visible, and infrared frequencies. The use of IRIS as a thermometer in space enables more accurate atmospheric dynamic models [3]. This aids the future of space missions, particularly initiatives to find habitable environments for alien life. Although IRIS shut down in 1988, it transmitted important information about Neptune and Uranus which led to our understanding that the two planets have roughly the same temperature. Since Neptune is substantially further away from the Sun, scientists concluded that the similarity in temperature was due to higher methane content trapping more heat on Neptune.
Voyager 1 has the Golden Record: a message from humanity to intelligent alien species including greetings in 55 human languages, pictures of people and places on Earth, and various samples of music. The two sets of Golden Records spin at 16.67 RPM and play a variety of famous songs from classical Mozart to popular American 1970s songs along with various sounds of nature, humans speaking, and other naturally occurring sounds from the planet. The Golden Record also shows a pulsar map to guide any viewers toward Earth [4]. Pulsars are the remains of dying stars whose rapid spinning and magnetic field emit a beam of light, which is why pulsar diagrams show planets around their star connected with beams of light. Figure 1 depicts the Golden Record as presented on the NASA website.
The spacecraft also had an Optical Calibration Target, a flat rectangle with constant color and light properties for cameras and infrared instruments on the spacecraft to calibrate themselves [5]. The Optical Calibration Target served as a visual target with known properties to compare measurements from space, particularly since different cameras and sensors aboard the Voyager satellites had their own characteristics and exhibited different pictures/visual patterns.
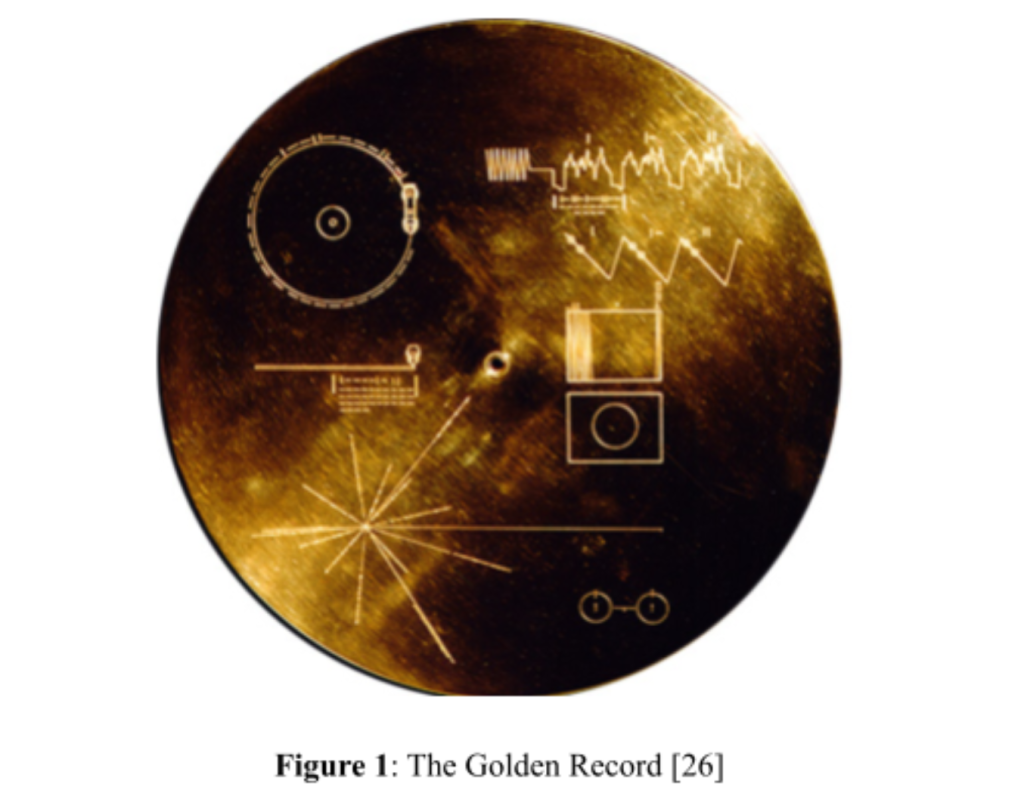
Launch Vehicle and Orbit
Voyager’s launch and path is something of a beauty itself, designed to take advantage of an arrangement of planets which only occurs every 175 years. Voyager swung from planet to planet in a process called gravity assist, because the gravitational pull of each planet (Jupiter, Saturn, Uranus and Neptune) bends the flight path and increases its speed enough to swing it to the next destination. This process minimized the propellant and need for engines and cut the flight time to Neptune from 30 years to 12 years.
Gravity assist lies on satellites orbiting about space bodies in an hyperbolic shape, thus conducting their flybys of planets at periapsis. The hyperbolic orbit is determined by the magnitude of the force of gravity acting on the satellite from the planet or moon it orbits. Since energy is conserved in an orbiting system, and periapsis means that the satellite is the closest to the planet as it will ever be, satellites fly very quickly past their planets [6]. The science equipment and data gathering features onboard then have very little time to actually image and collect data about the planets they fly by.
Launching a deep space vehicle like Voyager requires a period of powered flight which carries the vehicle from Earth’s atmosphere to free flight in space. This requires a launch vehicle to thrust the satellite into orbit which eventually falls off at burnout when the satellite is in free flight. In flight, Voyager 1 focused on flybys of Jupiter, Saturn and Titan, Saturn’s largest moon. Voyager 2 continued from Saturn and Jupiter to Uranus and Neptune as well. Both Satellites are now in deep space beyond the solar system, and it is anticipated that they will continue sending information back to Earth for 20-30 years. They are the longest flying and farthest venturing spacecraft in history and provide scientists with valuable information about the nature of energy and radiation in space [7].
Deep Space Networks
Sharing data from deep space satellites like Voyager back to Earth requires precise time synchronization. This is accomplished by the use of Deep Space Networks (DSNs) in California, Australia and Spain which provide coverage for all space missions [6]. Communication with DSN technology requires precise time information because while in orbit, there are times when a satellite cannot directly communicate with Earth. Whether the sun is between Earth and the satellite, or the body that the satellite is orbiting interferes in the signal, DSN systems can only transmit signals at allocated time intervals. To do so, NASA had used a manual system initially but has now adopted a digital, Sequence of Events (SOE) driven automation system. The SOE for Voyager contains information about the state of the spacecraft, any deviance/changes to the flight path, notification for the beginning and end time of orbits, information about the bit size and format of data being transmitted, and changes in the frequency of information being transmitted [8].
Pioneer
Pioneer 10, launched in March 1972, was built to study Jupiter’s atmosphere, the magnetosphere and moons around Jupiter like Io in particular. Pioneer 10 was the first satellite to fly beyond the asteroid belt, and also collected data on the solar wind patterns and dust distribution around Jupiter. Pioneer 10 completed its flyby of Jupiter in 1973 and became the first spacecraft to ever cross the solar system into interstellar space. Due to a failure of the power source, Pioneer 10 stopped transmitting information to Earth in 2003 but its 30 year flight far exceeded the 21 month mission it was assigned for. It is flying with a trajectory to reach the star Aldebaran in roughly two million years [9]. Pioneer 11 was the twin spacecraft of Pioneer 10, launched in April 1973 to primarily study Saturn although it passed Jupiter in gravity assist. Although Pioneer 11 experienced a number of malfunctions like the temporary failure of the Radioisotope Thermographic Generator, the heat and power source, the mission was a success and significantly enhanced human understanding of Saturn [10].
Science of the Mission
Pioneer 10 and 11 carried a tool called a helium vector magnetometer (HVM) which measured magnetic fields around Jupiter. The NVM protruded ~6.5 meters out from the rest of the satellite to reduce the effects of the spacecraft magnetic field and balance the spin of the spacecraft. Scientists had previously been able to observe the interplanetary magnetic partners from orbits around Mars, and they remained curious about the impact of the Sun’s magnetic field controlling the flow of plasma and magnetic energy to the rest of the solar system. With the Pioneer missions, the HVM collected data on the structure of the interplanetary field up to Jupiter, mapped a specific magnetic field of Jupiter itself and evaluated solar wind interactions about Jupiter [11].
Pursuant to the mission to better understand the Sun’s impact deep in our solar system, Pioneer carried a Cosmic Ray Telescope (CRT) to monitor solar rays and track high energy particles from the Sun. The device also measured particles in Jupiter’s orbit. It was composed of three telescopes, one for high energy particles, another for medium energy, and a last for low energy particles [12]. Coupled with data from future missions including Voyager, this data has led to a more precise understanding of Jupiter’s energy and how the Sun’s energy fades at further points in the solar system.
Pioneer was also the first satellite to take detailed pictures of the Great Red Spot, a massive storm on Jupiter larger than Earth. Pioneer 10 made observations of Jupiter’s poles and enabled a much more detailed perception of Jupiter to Earth’s population.
Launch Vehicle and Orbit
Pioneer 10 was the first spacecraft to use gravity assist to reach escape velocity to leave the solar system. By slingshotting from Jupiter to Saturn, Uranus and Neptune, Pioneer 10 escaped on its trajectory into interstellar space and was the furthest human built device from Earth, until it was surpassed by Voyager in the late 1990s.
When Pioneer was launched from Earth, it entered a heliocentric orbit which was tweaked with small thrust maneuvers from the Pioneer Navigation Team until the gravitational field strength of Jupiter exceeded that of the Sun. After the flyby of Jupiter, Pioneer returned to a heliocentric orbit until it reached Saturn [8]. A paper from researchers at the Jet Propulsion Laboratory about the anomalies detected in Pioneer’s orbit also includes an informative image of the paths taken by both Pioneer missions (see Figure 2).
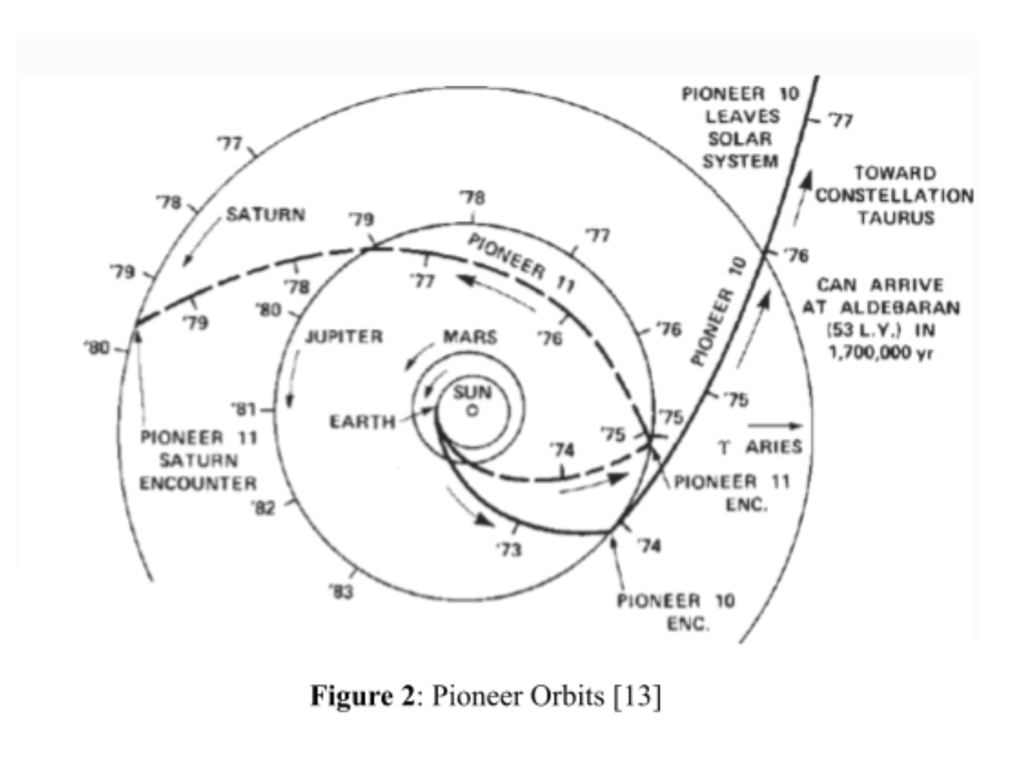
Pioneer 10 and Pioneer 11 Distinctions
Both Pioneer spacecraft were created almost identical and were simply launched with different intended orbits to research different bodies. Pioneer 11 last transmitted data in 1995 and Pioneer 10 last transmitted data in 2003. Both spacecraft served long after they were anticipated to fail and data from the outer brinks of the solar system lead to an understanding of a discrepancy between the anticipated speed of travel and the real speed dubbed the Pioneer Anomaly. The primary science difference between the two mission lies in their trajectory: Pioneer 10 explored Jupiter, its magnetic field and its satellites in great detail, then flew deeper into the solar system while Pioneer 11 hyperbolically sped past Jupiter, still capturing data and images, primarily aiming for Saturn and research on Saturn’s rings, magnetic fields and properties [10]. Pioneer 11 is now hurtling towards the center of the Milky Way although humanity lost contact with it decades ago. The Golden Record is still prominently shown on both spacecraft for any curious intelligent life in the future.
Pioneer Anomaly
Years into both Pioneer flights, it was noticed that the spacecraft were not moving with the acceleration that was predicted. On the outer edges of the solar system, both Pioneer spacecraft slowed down (5000 km per year). This deviation in behavior initially caused concern in the physics community, because without a reasonable explanation, the Newtonian models of physics would have to be questioned. Eventually, Doppler data (information about lightwaves of different wavelengths) were transmitted from Pioneer back to Earth which set the record straight. The reason for the slow down was simply the anisotropic dispersion of the internal heat generated from the science tools onboard each spacecraft. A paper from JPL describes the issue, saying that “Pioneer spacecraft were powered by SNAP-19 (Space Nuclear Ancillary Power) RTGs mounted on long extended booms designed to protect the on-board electronics from heat and radiation impact”. When these SNAPs got hot, the thermal radiation produced could disrupt the balance of forces on Pioneer, but NASA had placed them on specially balanced rods far away from the body of the spacecraft in order to balance each other out. The failure of this system is currently being researched and remains a topic of interest to amend for future missions. Since the balancing mechanism did not work, SNAP emitted thermal photons in an uneven way, which in return caused a recoil force in the opposite direction of the photos. After all, momentum is still conserved within the photon-spacecraft body. This uneven distribution of force is the current accepted explanation for the discrepancy between anticipated and real speed [13].
Galileo
NASA’s Galileo mission was a historic first for human exploration in space and led to an immense growth in understanding Jupiter. Galileo was the first satellite to ever orbit an outer planet, and it spent eight years orbiting Jupiter gathering information on the planet itself and its various moons. Data gathered from Galileo is the basis for future missions to Europa to explore the potential of subsurface water, among other future space missions as well.
Science of the Mission
Galileo used plasma and particle detectors like the Voyager and Pioneer missions, but employed a much more sophisticated set of cameras and sensors for near-Jupiter orbit.
The Solid State Imaging Camera (SSI) was intended to study Jupiter and its satellites using a modified spare of the narrow angle telescope used on Voyager. The camera operated in eight filtered band passes and provided higher resolution, better filters and more effective bandwidth. Although the SSI did not come with an ultraviolet band, the images were clearer and more useful than prior missions. The amended design from the Voyager mission also improved performance in baffling, a process which eliminates visual noise from scattered light. The primary science missions of this advanced camera was to investigate Jupiter’s atmosphere and clouds, measure and determine the properties of Jovian satellites, map the distribution of minerals on the surface of Jupiter’s moons and search for any other behavior or atmospheric emissions, particularly from the night side of Jupiter [14].
Galileo also carried the Heavy Ion Counter (HIC), an engineering experiment to monitor heavy ion activity in order to find information on radiation near Jupiter and determine the parameters for designing future radiation safe electric material for deep space exploration. The experiment included two Low Energy Telescopes (LETs) used to sample a wide range of energies. The information gathered from Galileo, when combined with data from Pioneer and Voyager, has offered important guidance to scientists about the future of electricity and radiation in space.
Since the Galileo mission was orbiting around Juptier and could not escape further into deep space, it was instructed to hurtle towards Jupiter’s atmosphere in 2003 where it was destroyed. The mission was over because it had collected enough relevant data for NASA to use. Galileo also took a number of detailed pictures of Jovian satellites, including the moon Io (see Figure 3).
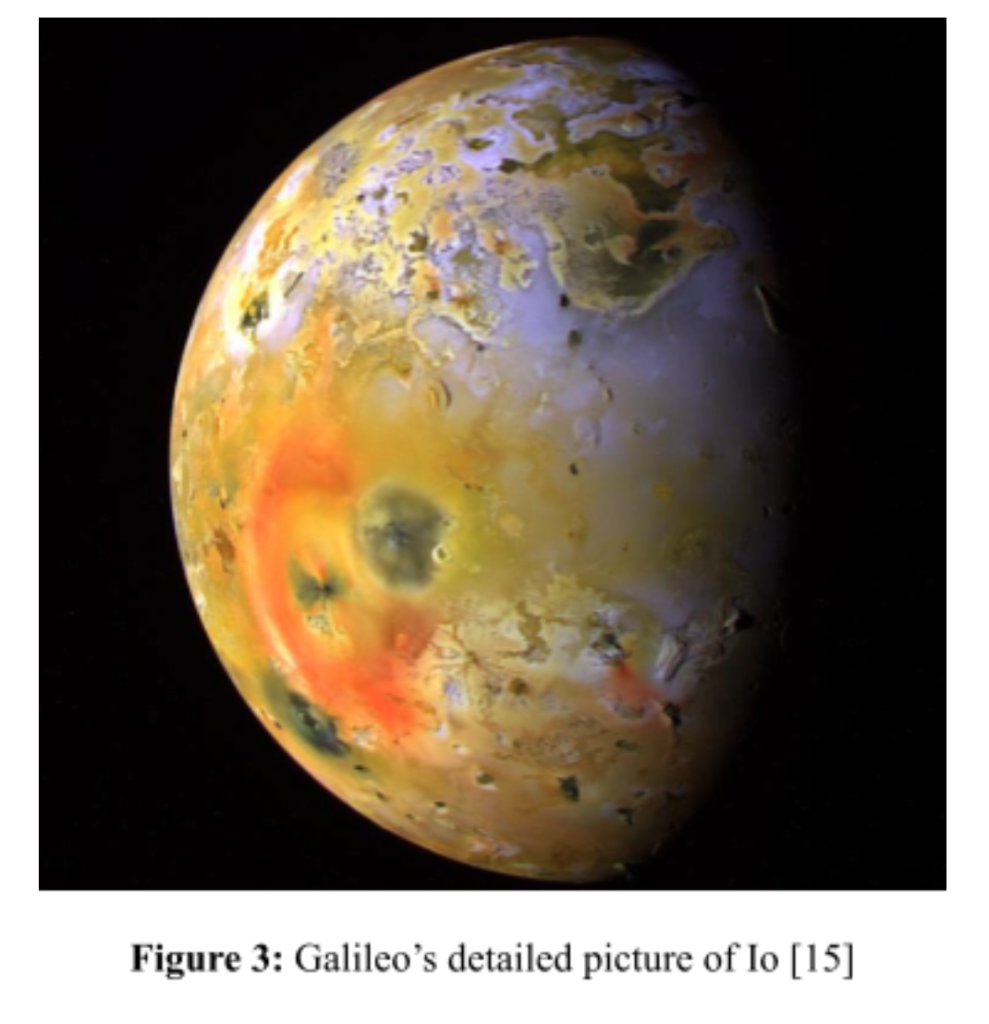
Launch Vehicle and Orbit
Galileo was the first mission to ever orbit an outer planet. To do so, the spacecraft had to use gravity assist from Venus and Earth to pass the asteroid belt and reach Jupiter. Galileo did one flyby of Venus and one of Earth, gathering enough momentum from the hyperbolic path it traced around both to thrust itself towards Jupiter. Along the way, Galileo even took pictures and gathered data of a few large bodies in the asteroid belt. Galileo was composed of a spacecraft and a probe. The spacecraft was the source of thrust around other bodies while the probe was intended to be a short-lived tool descending in the atmosphere to measure temperature and other atmospheric factors. Six years after leaving Earth, Galileo reached Jupiter and the probe separated from the spacecraft, entering Jupiter’s atmosphere. The probe descended for just under an hour with parachutes and transmitted data on the strong winds, intense heat and unexpected dryness of the Jovian atmosphere before it was vaporized by the head [15].
While the spacecraft relayed this information from the probe back to Earth, mission scientists at NASA had to determine the right moment to activate the engine on the spacecraft to enter Jupiter’s orbit. Too little thrust, and Galileo would sail past Jupiter into deep space, and too much thrust would force the spacecraft to the same short lived life as the probe: melted in the atmosphere. The decisions made were successful and Galileo entered orbit, where it remained for two years. Galileo maintained a long elliptical orbit around Jupiter because the different distances away from the planet enabled the devices onboard to gather data on the planet, the rings around it and conduct flybys of important moons [16].
The Galileo spacecraft and probe were launched by an Inertial Upper Stage (IUS) launch vehicle. IUS is an unpiloted booster used from the 1980s to the early 2000s. After launching from the ground, the IUS employed a second stage launch boosting its payload into higher orbit, or in Galileo’s case, to another planet of the solar system. The IUS was designed by Boeing and the two rocket burns happened one hour, then six and a half hours into flight [17].
Cassini
Cassini was a joint mission between NASA, European Space Agency and the Italian Space Agency to explore Saturn, its moons and its rings. Much like Galileo, Cassini employed a probe called Huygens to explore Titan, Saturn’s largest moon, while a spacecraft orbited Saturn gathering other data.
Science of the Mission
The science devices onboard the Cassini orbiter fall into three categories: Optical Sensing, Fields/Particles and Microwave Sensing.
With regards to Optical Sensing operations, Cassini carried a Visible and Infrared Mapping Spectrometer (VIMS) which collected light that is visible to humans, and infrared light of longer wavelengths. VIMS separated light by the wavelengths it detected which allowed scientists to break down the composition of Saturn’s atmosphere, its rings and its satellites as well. This proved important during orbit because NASA determined that Titan has an ice volcano, and Titan also had clear polarity similar to Earth with cold poles and a warmer center. While VIMS was used to disassemble specific views on Saturn, Cassini also carried an Imaging Science Subsystem (ISS) which functioned as the main eyes for the spacecraft. While VIMS focused on the visible spectrum and infrared light, ISS also caught ultraviolet light [18]. The ISS made Cassini famous both due to its role in navigation and because most of the iconic pictures from Cassini came from ISS. Popular images of Saturn and its rings came from the ISS, along with important data about the surface of Titan. This information came from blocking all but certain wavelengths to see below the atmospheric smog.
To better conceptualize the plasma, electric/magnetic fields and energy systems around Saturn, Cassini also carried a Magnetometer (MAG) and the Cassini Plasma Spectrometer (CAPS). MAG was a simple device similar to tools used in other missions. While the Cassini spacecraft orbited Saturn, it recorded the direction and strength of magnetic fields around the spacecraft to develop a 3-D model of the magnetosphere and understand how it impacts the moons, the rings and gasses around Saturn. Thanks to Cassini’s elliptical path around Saturn, it orbited in and out of the magnetosphere which allowed a more sophisticated model to be built based on observations from inside and outside the zone of interest. Going deep into Saturn’s dense helium core would yield more information as well, but remains a figment of imagination right now because any human built spacecraft would vaporize well before getting to the center of Saturn. Much like the Voyager spacecraft, having a magnetic detector right next to a metal body would significantly skew results, so MAG was actually installed on an 11 meter metal arm as far away from the spacecraft as possible [19]. This arm was folded at takeoff and simply opened two years after it left Earth. CAPS had three sensors: an electron sensor, ion mass spectrometer and ion beam sensor. Each of them measured particle kinetic energy and direction, but the ion mass spectrometer collected data on particle mass as well [20]. Yet again thanks to an elliptical orbit which enabled the spacecraft to interact with Saturn’s atmosphere at different radii from the surface of the planet, CAPS was able to collect information about the composition of the atmosphere and magnetosphere
To collect information on microwave activity, Cassini also carried the Radio Science Subsystem (RSS) which sent its own radio signals towards Saturn and learned about the objects it interacted with by the radio waves which bounced back towards Cassini. This was used to learn about gravity fields, the structure of the rings of Saturn and some of the surface properties as well [21]. Coupled with the visual data from the cameras and the atmosphere information from the probe, this led to a much more complete model of Saturn and deeper understanding of the rings and satellites around it.
Launch Vehicle and Orbit
Cassini’s orbit is a work of gravity and geometric art. While the path appears to be elliptical, Saturn is actually acting much more like a focus point for the orbit. That is to say, Saturn is relatively close to the end of the major axis of the ellipse. In reality, the orbit of Cassini has been a combination of two hyperbolic traces: a choreography between Saturn and Titan, a massive moon. Cassini begins its journey towards Saturn, crossing between the planet and its rings, when it is the furthest away from Saturn: a point known as apoapse. After that point, Cassini swirls around and away from Titan towards Saturn. Prior to the Cassini mission, Titan was only photographed by Voyager and observed from Earth [22]. These close, hyperbolic orbits not only enabled more detailed photography and research, but the Huygens probe was able to get to the surface of Titan and gather important information.
Cassini was guided to space by the Titan IV-B launch vehicle, which was NASA’s most powerful launch vehicle at the time employing a two stage launch system. The Titan series had been used by the Air Force for various military applications, and were employed extensively by NASA due to their reliability and sheer power [23].
Conclusion and Future Missions
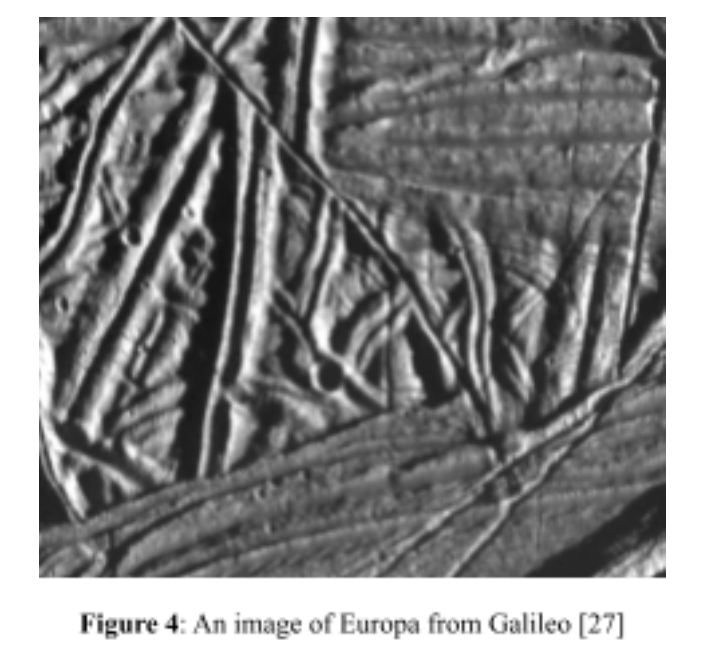
Right now, NASA has dozens of plans to conduct flybys of important asteroids and planets in the coming years. Venus and Mercury seem to be of particular interest, which makes sense given the past focus on Jupiter and Saturn. While the space community believes firmly in the importance of such initiatives, and supports the continued research of planets and space bodies, there is interest in the Europa Clipper mission scheduled for 2024 [24]. Prior images of Europa from NASA show a fascinating fractured surface (see Figure 4) which appears interesting to study. The plan is for NASA to send a spacecraft into Jupiter’s orbit in order to conduct numerous fast flybys of Europa, likely employing the same broadly elliptical shaped orbit around Saturn. Given that Europa is known to have icy surfaces and might harbor water, there is also value in sending a probe to Europa’s atmosphere to better understand its habitability. The same techniques from Galileo can be employed and the information from a probe coupled with a spacecraft would likely yield more direction for future missions than just distant observations from a spacecraft [25].
The exploration of space was unthinkable 100 years ago and has become a reality today. Deploying principles like gravity assist, using hyperbolic flybys of planets and manipulating energy across our solar system has enabled humanity to access and explore worlds previously only dreamt of. As the advent of a period of continued space exploration dawns and humanity prepares to take off on the path to understanding the universe around us better, it is important to recall the physics and engineering concepts discovered and proven in past missions while embracing the future of innovation and change to come.
Bibliography
[1] “Voyager – The Interstellar Mission,” voyager.jpl.nasa.gov. http://voyager.jpl.nasa.gov/mission/interstellar-mission/
[2] J. W. | P. Thursday, October 24, and 2019, “40 years later, these Voyager instruments still talk to NASA,” Astronomy.com, Oct. 29, 2019. https://astronomy.com/magazine/2019/10/after-40-years-voyager-still-talks-to-nasa-with-7 -instruments
[3] “Voyager – Spacecraft – Infrared Interferometer Spectrometer and Radiometer (IRIS),” voyager.jpl.nasa.gov. https://voyager.jpl.nasa.gov/mission/spacecraft/instruments/iris/
[4] J. Lee Oakes, “Voyager Golden Record: Through Struggle to the Stars,” Smithsonian Music, Sep. 24, 2019. https://music.si.edu/story/voyager-golden-record-through-struggle-stars
[5] “Ring-Moon Systems Node – Voyager 1 Narrow Angle Camera Description,” pds-rings.seti.org. https://pds-rings.seti.org/voyager/iss/inst_cat_na1.html (accessed Nov. 20, 2022).
[6] R. Braeunig, “Basics of Space Flight: Orbital Mechanics,” www.braeunig.us. http://www.braeunig.us/space/orbmech.htm#launch (accessed Nov. 20, 2022).
[7] NASA, “Voyager 3D Model,” NASA Solar System Exploration, Jul. 26, 2019. https://solarsystem.nasa.gov/missions/voyager-1/in-depth/
[8] R. Hill, S. A. Chien, C. Smyth, K. Fayyad, and T. Santos, “Planning for Deep Space Network Operations,” undefined, 1995, Accessed: Nov. 20, 2022. [Online]. Available: https://www.semanticscholar.org/paper/Planning-for-Deep-Space-Network-Operations-Hill -Chien/64e8702993baffc32f911272ba1122a91bbd3ba3#citing-papers
[9] “The Outer Planets: Missions: Pioneer 10 & 11,” lasp.colorado.edu.https://lasp.colorado.edu/outerplanets/missions_pioneers.php
[10] “Pioneer 10 and 11, outer solar system explorers,” The Planetary Society. https://www.planetary.org/space-missions/pioneer
[11] “In Depth | Pioneer 10 – NASA Solar System Exploration,” NASA Solar System Exploration, Jul. 24, 2019. https://solarsystem.nasa.gov/missions/pioneer-10/in-depth/
[12] F. McDonald, “Cosmic Ray Telescope Experiment (CRT), Pioneer 10/11 Program,” Technical Report, Maryland Univ. College Park, MD United States Inst. for Physical Science and Technology, Jun. 1999, Accessed: Nov. 20, 2022. [Online]. Available: https://ui.adsabs.harvard.edu/abs/1999STIN…9964263M/abstract
[13] S. G. Turyshev and V. T. Toth, “The Pioneer Anomaly,” Living Reviews in Relativity, vol. 13, no. 1, 2010, doi: 10.12942/lrr-2010-4.
[14] “NASA – NSSDCA – Experiment – Details,” nssdc.gsfc.nasa.gov. https://nssdc.gsfc.nasa.gov/nmc/experiment/display.action?id=1989-084B-10#:~:text=Des cription
[15] “Galileo – In Depth,” NASA Solar System Exploration, Aug. 15, 2018. https://solarsystem.nasa.gov/missions/galileo/in-depth/
[16] “Galileo Project Information,” nssdc.gsfc.nasa.gov. https://nssdc.gsfc.nasa.gov/planetary/galileo.html
[17] “Boeing Inertial Upper Stage Space Payload Booster,” National Museum of the United States Air ForceTM. https://www.nationalmuseum.af.mil/Visit/Museum-Exhibits/Fact-Sheets/Display/Article/6 93053/boeing-inertial-upper-stage-space-payload-booster/#:~:text=The%20Inertial%20Up per%20Stage (accessed Nov. 20, 2022).
[18] “Imaging Science Subsystem (ISS) | Cassini Orbiter – NASA Solar System Exploration,” NASA Solar System Exploration, Sep. 04, 2018. https://solarsystem.nasa.gov/missions/cassini/mission/spacecraft/cassini-orbiter/imaging-science- subsystem/
[19] “Magnetometer (MAG) | Cassini Orbiter,” NASA Solar System Exploration. http://solarsystem.nasa.gov/missions/cassini/mission/spacecraft/cassini-orbiter/magnetome ter/ (accessed Nov. 20, 2022).
[20] “Cassini Plasma Spectrometer (CAPS) | Cassini Orbiter,” NASA Solar System Exploration. http://solarsystem.nasa.gov/missions/cassini/mission/spacecraft/cassini-orbiter/cassini-plas ma-spectrometer/ (accessed Nov. 20, 2022).
[21] “Radio Science Subsystem (RSS) | Cassini Orbiter – NASA Solar System Exploration,” NASA Solar System Exploration, Sep. 04, 2018. https://solarsystem.nasa.gov/missions/cassini/mission/spacecraft/cassini-orbiter/radio-scien ce-subsystem/
[22] “Ring-Grazing Orbits,” NASA Solar System Exploration, Apr. 11, 2017. https://solarsystem.nasa.gov/news/12966/ring-grazing-orbits/ (accessed Nov. 20, 2022).
[23] “Remember the Titans,” Lockheed Martin, Jun. 15, 2022. https://www.lockheedmartin.com/en-us/news/features/history/titan.html
[24] “Upcoming Events,” nssdc.gsfc.nasa.gov. https://nssdc.gsfc.nasa.gov/planetary/upcoming.html
[25] “Europa Clipper,” www.jpl.nasa.gov. https://www.jpl.nasa.gov/missions/europa-clipper [26] NASA, “Voyager – The Golden Record Cover,” Nasa.gov, 2019. https://voyager.jpl.nasa.gov/golden-record/golden-record-cover
[27] “Ridges and Fractures on Europa,” NASA Solar System Exploration, Mar. 28, 1998. https://solarsystem.nasa.gov/resources/105/ridges-and-fractures-on-europa/?category=miss ions_galileo (accessed Nov. 20, 2022).
About the author
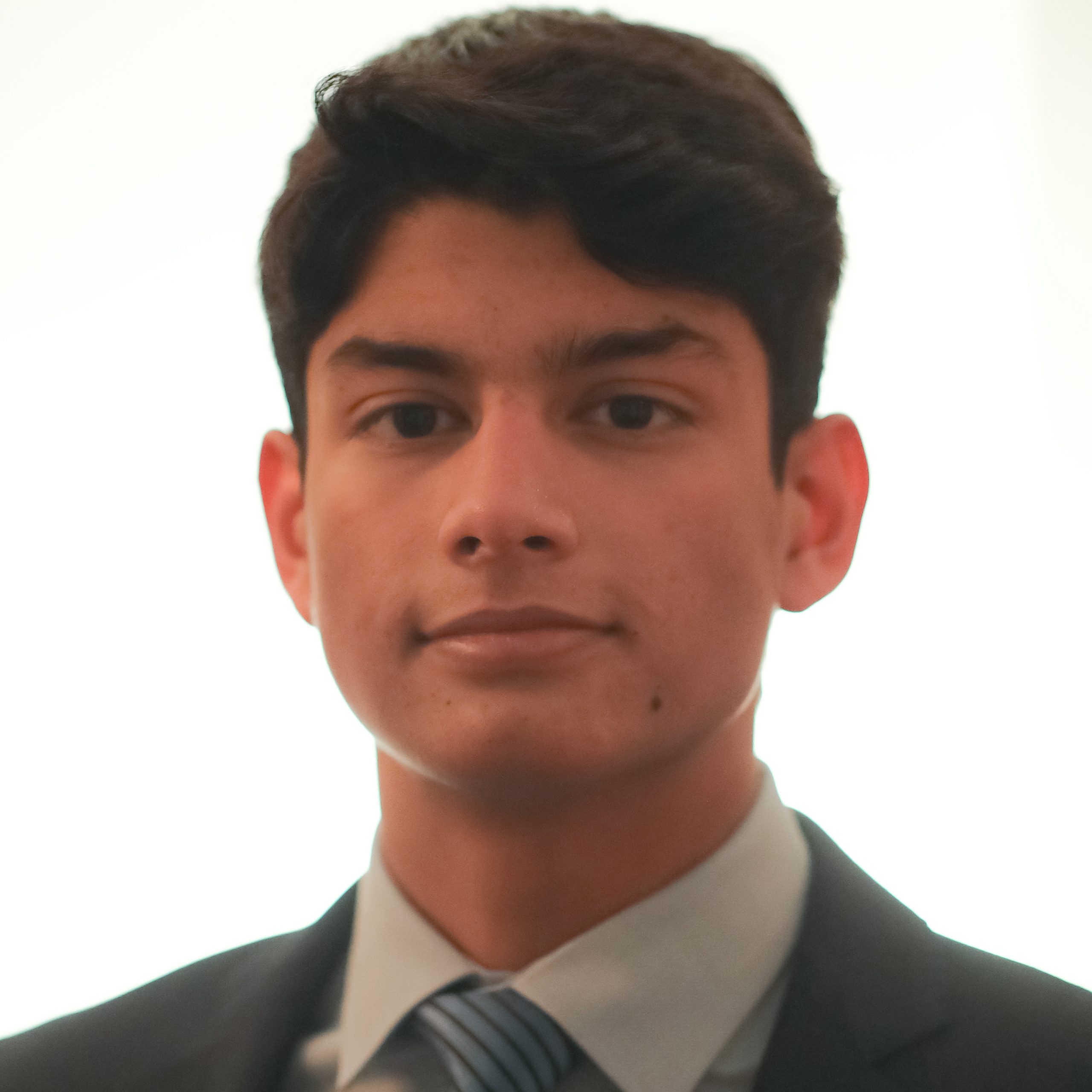
Aniket Martins
Aniket is a senior at Fairfield Ludlowe High School in Fairfield, CT. He is passionate about aerospace engineering and is a member of the American Institute of Aeronautics and Astronautics where he led the 2022 Congressional Visits Day lobbying initiatives for Connecticut. He participated in the University of Florida’s Student Science Training Program in 2022 where he interned at the Nanostructured Energy Systems Lab, completing a research project in Applications of Microfluidic Coolant Systems in Atmospheric Reentry Temperature Regulation for which he won Best Presentation.
Aniket is also passionate about civics and is President of his school’s award winning Model UN team and has won numerous awards at various conferences. At his school he is also President of Mu Alpha Theta Math Honor Society, Vice-President of National Honor Society, Vice-President and Director of Tutoring for English Honor Society, President of Rocketry Club, Founder/President of Engineering Club and an editor for Prospect, the school newspaper. Aniket has won numerous awards in the Connecticut Writing Contest and loves soccer, skiing and tennis.