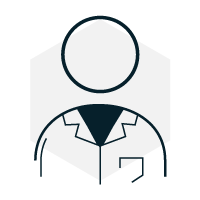
Author: Madalyn Shen
Mentor: Cesar Terrer
Germantown Friends School
Abstract
Mycorrhizae are symbiotic relationships between plants and fungi in which the fungi supply the plant with water, nutrients, and other beneficial substances to maximize their growth, and receive carbohydrates and organic compounds by the plant’s ability to photosynthesize. Arbuscular mycorrhizal fungi (AMF) are the most abundant type of mycorrhizae and is the most well-researched. Agriculture is harmful for the climate and the environment, and projections in human population will only increase the pressures of agriculture. The topic of mycorrhizae has suddenly attracted the interest of many scientists and intense studies because of its potential benefits in croplands, which could reduce fertilizer use (reducing greenhouse gas emissions) and increase crop yields (increasing food security). However, because the subject has recently been brought about, not enough research has been conducted to confirm the mechanics, benefits, and potential utility of AMF in crop plants. This study offers a detailed and unified view of two of the most important benefits of mycorrhizae in various crops: its ability to increase water and nutrient uptake and to enhance resistance to abiotic stress.
Introduction
Mycorrhizae is a symbiotic association between plants and fungi. Plants produce organic compounds, such as carbohydrates, by photosynthesis and supply them to fungi through root tissues. In return, fungi supply water and nutrients to plants through their high absorptive ability because of the large surface area of fungal hyphae (Selosse et al. 2006). In some areas, such as lowland forests, fungi can create a network between all trees and use them to exchange nutrients (Read and Perez-Moreno 2003). There are four types of mycorrhizae: arbuscular mycorrhiza, orchid mycorrhiza, ectomycorrhiza, and ericoid mycorrhiza. Arbuscular mycorrhizal fungi (AMF) are the most common fungi in soils, representing up to 55% of the soil microbial biomass and forming a symbiosis with more than 80% of vascular-cultivated plants (Diagne et al. 2020). AMF are natural root symbionts that provide essential inorganic nutrients to host plants, thereby improving growth and yield under various conditions (Begum et al. 2019). As a biofertilizer, AMF can potentially benefit plants in many ways, thereby playing a vital role in agricultural ecosystems.
It is becoming increasingly crucial to supply food to a growing human population while mitigating the effects of climate change. The world’s population is more than three times larger than that of the mid-twentieth century. The global human population has reached 8 billion by 2022, 5.5 billion more than in 1950. The world’s population is expected to increase by nearly 2 billion people in the next 30 years, from the current 8 billion to 9.7 billion in 2050 and could peak at nearly 10.4 billion in the mid-2080s (Roser and Rodés-Guirao, 2013). A greater population leads to greater demand for food, water, and natural resources. Agriculture accounts for 10-15% of greenhouse gas emissions (Houser and Stuart 2020). Nitrogen fertilizers, popular chemical fertilizers used to grow crops today, emit large amounts of greenhouse gases. This occurs when the fertilizer runs off into waterways or is broken down by soil, thereby releasing nitrous oxide gas. Population growth and greenhouse gas emissions are among the reasons why the study of mycorrhizae is important.
Because mycorrhizae are a relatively new subject, little research has been conducted on this topic, and agricultural and environmental ramifications are unclear. Field studies have shown that AMF increase nitrogen uptake in wheat crops by 12.9% (Giambalvo et al. 2022) and onion crops by 75% (Mollavali et al. 2018). It is unclear why results differ greatly between different crop plants, and the fact that experiments and studies only target one type of crop, or one aspect of mycorrhizae does not help. There is much ambiguity surrounding the fascinating idea of using mycorrhizae to increase crop yields, which should not be the case, since there are many clear benefits. Much research is needed to identify how impactful mycorrhizae can be in the field of agriculture and in the face of climate change. In this paper, I discuss the benefits of mycorrhizae in increasing water and nutrient uptake and plant tolerance to abiotic stress.
1. Mycorrhizal benefits in water and nutrient uptake
Mycorrhizae increase water uptake through their wide network of filaments, which gives the plant a larger soil contact surface area than they can reach alone (Huey et al. 2020). Collectively, the extremely fine threads of mycorrhizal hyphae, a long and branching filament structure of a fungus, act as a sponge to absorb water when it becomes available and holds onto it for times of need (“Water Acquisition” n.d.). In other words, they act as water reservoirs for plants and increase their ability to absorb water. This factor will emerge later as we discuss the benefits of mycorrhizae in increasing the tolerance of plants to environmental stress. The absorptive area of mycorrhizal hyphae is approximately 10 times more efficient than that of root hairs, with the longest area reaching 10 cm (Huey et al. 2020). In an experiment performed by J. M. Ruiz-Lozano and R. Azcón, the two growing heads of lettuce, were placed in separate compartments divided by a mesh. One compartment contained only the roots. The other specimen had AM hyphae. Water was accessible to both plants because of the steel mesh. The results indicated that more water was taken up by the compartment with AM (Ruiz-Lozano and Azcón 1995), indicating their superior absorptive abilities and water uptake.
Mycorrhizae increase phosphorus uptake. Because phosphorus dissolves in water, mycorrhizae indirectly take up phosphorus through hyphae when taking in water for the plant. However, inorganic phosphorus is scarce in the soil because it has a low diffusion rate, is sequestered by minerals like iron and aluminum hydroxides, and is bound to organic matter (Lambers, Martinoia, and Renton 2015). The availability of phosphorus is further compromised by geological aging and weathering in the soil (Clausing and Polle 2020). Plants adjust to varying phosphorus availability by adopting root systems that explore organic soil more intensely in phosphorus-poor soil than in phosphorus-rich soil (Lang et al. 2016). Mycorrhizae send signals to plants, such as LjPT4 and MtPt4, which induce a genetic process by which the plant is more effective in absorbing phosphorus (Posta et al. 2019). Some studies have shown that AMF can contribute up to 90% of the plant phosphorus uptake (van der Heijden et al. 2015).
Mycorrhizae can also increase nitrogen uptake. Nitrogen can be present in either organic or inorganic forms in soil. Plants require inorganic nitrogen because, among other vital processes, it is essential for photosynthesis. The main role of mycorrhizae is to access more inorganic nutrients, such as nitrogen. The mycorrhizal sheaths of the trees contributed 50% or more of the total nitrogen input into the Douglas fir ecosystem (Read and Perez-Moreno 2003). Similarly, Swedish coniferous forest soils contained up to 20% of the total nitrogen in a given horizon that could be located in the fungal mycelium (Read and Perez-Moreno 2003). The evolution of saprotrophic ancestors, fungi that obtain nutrients by feeding on organic matter, plays a major role in the ability of fungi to obtain inorganic nitrogen (Johnson and Gehring, 2007). By adapting their metabolism to the availability of nutrients in the soil, fungi are able to produce a mixture of oxidative and hydrolytic enzymes to break down lignocelluloses, such as wood (“Fungal Extracellular Enzyme Activity” 2023). The fungi release the enzymes into the surrounding soil, which consume organic matter, such as decomposing wood, and turn it into inorganic nitrogen in a process called nitrogen mineralization. This process is crucial to nutrient uptake in plants, as it cannot trigger this chemical reaction by itself. The fungi then trade inorganic fungi to the host plant to receive benefits, such as carbon and carbohydrates.
Additionally, mycorrhizae can reduce nutrient losses, a problem commonly overlooked in discussions of the ways mycorrhizae can bring in nitrogen, rather than how it could prevent it from being removed. Nutrient loss, especially in nutrient-poor ecosystems, reduces plant productivity and the overall integrity of the crops produced. Nutrient loss is primarily a result of leaching and loss of water-soluble plant nutrients due to rainfall and irrigation. This creates losses of up to 50-60% of all applied nitrogen (Melissa 2018). Grassland microcosms with AM fungi lost 60% less phosphorus and 7.5% less ammonium compared to control microcosms without AM fungi (van der Heijden 2010). Similar results were obtained for microcosms planted with three different grass species (van der Heijden 2010). Mycorrhizae prevent leaching in two ways: their widespread and complex root system and their enhanced nitrogen interception. As with water, increasing the absorptive surface of the root system allows AMF to explore a larger soil volume and access more nutrients (Asghari and Cavagnaro 2012). Studies have also shown that the regulation of key genes involved in nitrogen transport and assimilation indicates a shift towards nitrogen uptake via the mycorrhizal pathway in the mycorrhizal genotype (Asghari and Cavagnaro 2012). Further work is needed to test the effects of mycorrhizae on nutrient loss. Unfortunately, few studies have been performed under field conditions because of experimental constraints. Many experiments performed in controlled environments may yield biased and incomplete results. The combination of some of the studies that have been performed show that AMF increases nitrogen uptake by 54.3% and phosphorus uptake by 55-67-59.67%.
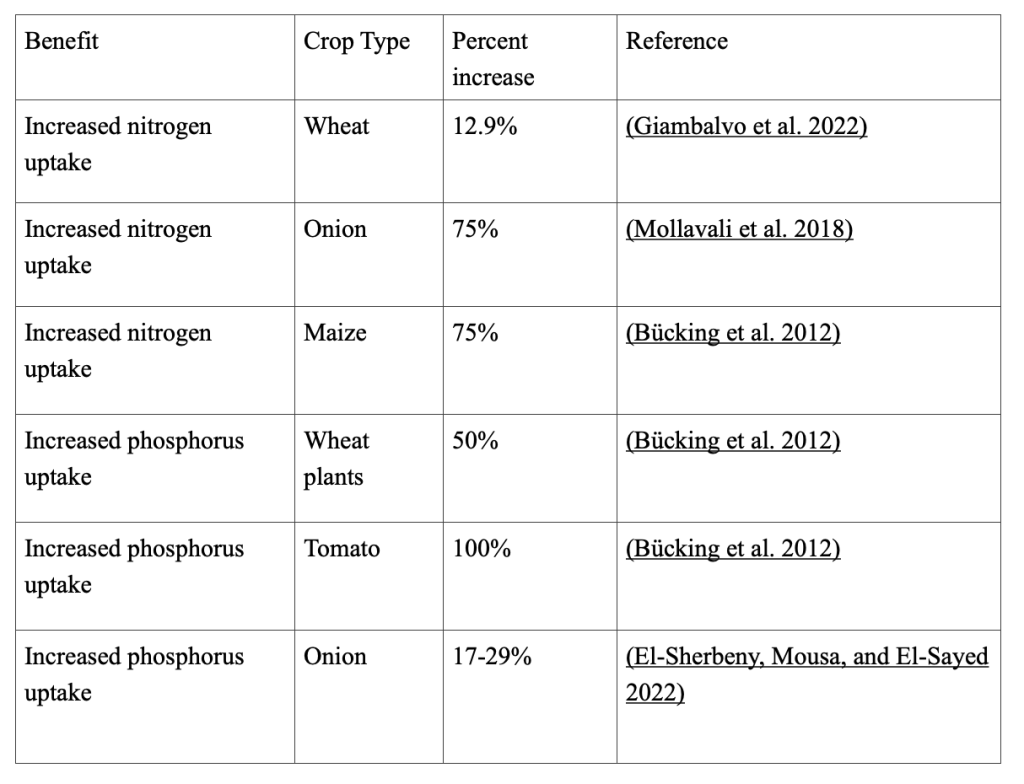
2. Mycorrhizal benefits in increasing plant tolerance to abiotic stress
Abiotic stress is the negative effect of non-living factors on living organisms, which hampers plant growth and productivity. These include drought, salinity, low or high temperatures, metals, and other environmental extremes (Stanca et al. 2003). Abiotic stress is further increased by climate change and agricultural malpractices such as the excessive use of nitrogen fertilizers and artificial pesticides. It is important to note that abiotic stress is much more genetically complex and more difficult to control than biotic stress, the condition in which plants cannot sustain their normal growth due to certain interferences from deleterious microorganisms, such as bacteria and viruses (Yahia 2019). Therefore, there is an increasing need for bio-fertilizers such as AMF, which are believed to provide tolerance to abiotic stress and prevent the decrease in key metabolic pathways in crop plants (Begum et al. 2019).
Water scarcity is the number one abiotic stress for crops in all soils, including arid, semi-arid, and even agricultural areas where crops are grown. Droughts have been further enhanced by climate change. A warmer temperature reduces surface water and dries out soil and vegetation. Droughts reduce plant nutrient uptake by decreasing both nutrients created by mineralization and nutrient diffusion throughout the soil (Bárzana and Carvajal 2020). Lack of nutrients negatively affects plant growth, crop yield, and quality. Over the past 35 years, worldwide droughts have decreased maize yields by 40% and wheat production by 21% (Posta et al. 2019). This is where mycorrhizae come in, or specifically, AMF. As discussed above, the hyphal network of AMF reaches a larger area of soil and can transport water through its fine filaments to the plant. Although this helps to provide the plant with water during droughts, another important factor is that AMF can improve the water-holding capacity of the soil. AMF produces glomalin or glomalin-related soil protein (GRSP), which binds to individual soil particles. GRSP enhances water retention in soils by protecting C-rich debris from decomposition by soil microbes (Posta et al. 2019). AMF hyphae interact with GRSP and physically stick microaggregates with macro-aggregates, which form stable aggregates under water-scarce conditions (Nichols 2008). In artificial substrates, host plants are less stressed in soil inoculated with AMF under drought conditions (Posta et al. 2019), which suggests that AMF increases a plant’s tolerance to water-scarcity stress by enhancing water retention in the surrounding soil.
Salinity, an increase in soil salt concentration, is another type of abiotic stress that is extremely degrading to crop plants. This problem is intensified by manmade irrigation systems in arid and semi-arid lands with low rainfall. Inadequate irrigation systems create salinity, which negatively affects 20% of all irrigated land (Glick et al. 2007). Salts in the soil occur as ions released by decomposing minerals in the soil. They can also be applied by irrigation and groundwater. When precipitation is insufficient to wash away these ions from the soil, salts begin to accumulate (Shrivastava and Kumar 2015). Although plants are able to absorb essential nutrients in the form of soluble salts, an excess of it simply overbears the root system, which leads to suppressed growth and other problems. Sodium is non-essential in most plants. However, excess potassium causes an ionic imbalance by interfering with potassium functions, which can lead to nutrient deficiency and the death of plant cells (Assaha et al. 2017). AMF can mitigate the effects of salt stress by maintaining a balance between sodium and potassium levels, which is key to survival in saline environments. AMF naturally form symbiotic relationships with plant species in saline environments (Juniper and Abbott 2006) and can improve the ionic balance between K and Na through selective uptake.
Another type of abiotic stress is caused by heavy metals, including Pb, Ni, Cu, and Hg. Heavy metals are toxic to plants and have been shown to suppress shoot and root growth, leaf chlorosis, and even death in soils enriched with cadmium and zinc (Moghadam 2016). Heavy metal contamination is one of the most serious hazards to agricultural ecosystems and human health because it is extremely common and non-biodegradable (Riaz et al. 2021). AMF mitigate the effects of heavy metal contamination by assisting phytoremediation and the use of microbes to reduce the concentrations of toxic substances in the environment (Yan et al. 2020). AMF-assisted phytoremediation is gradually gaining attention because it is an inexpensive and environmentally friendly technique with fewer secondary pollutant effects (Morar et al., 2018). Mycorrhizae can directly and indirectly mitigate the stress of heavy metals. Immobilizing heavy metals directly in the hyphae of mycorrhizal fungi can fix them in the cell wall and store them in the vacuole (Begum et al. 2019), thereby reducing metal toxicity in plants. In an experiment performed on alfalfa, AMF improved the tolerance of plants to Cd because of the immobilization of heavy metals (Wang, Huang, and Gao 2012). AMF can indirectly induce changes in host plants to cope with toxic environments by improving water and nutrient uptake (Riaz et al. 2021). As observed in Lolium perenne, or perennial ryegrass, AMF settle polluted soils and reduce the uptake and accumulation of heavy metals including cadmium, nickel, and zinc (Takács and Vörös 2005). In an experiment performed on rice, AMF was extremely effective in lowering the levels of Cd in both vacuoles and cell walls (Li et al. 2016). The many benefits of mycorrhizae decrease abiotic stress and allow crops to grow and prosper under extremely harsh conditions.
Conclusion
This study focused on the mycorrhizal benefits of increased water and nutrient uptake and increased resistance to different types of abiotic stress, including drought, salinity, and heavy metal toxicity. Again, because mycorrhizae are still a relatively new topic in the scientific field and have not been thoroughly researched, the agricultural and environmental consequences remain unclear. However, it is safe to say that it is definitely in the running for the key to pressing environmental issues. A compilation of the few experiments that have been performed show that AMF increases nitrogen uptake by 54.3% and phosphorus uptake by 55.67-59.67%, which is a huge indicator of its true potential. AMF is also proven to improve plant tolerance to droughts, salinity, and heavy metals toxicity. Perhaps gaining a wider and more complete view of the benefits of mycorrhizae will raise new questions that reach the heart of the problems at hand, such as how exactly we can apply the benefits of mycorrhizae to mitigate the effects of challenges we face today.
References
Asghari, Hamid R., and Timothy R. Cavagnaro. 2012. “Arbuscular Mycorrhizas Reduce Nitrogen Loss via Leaching.” PLOS ONE 7 (1): e29825. https://doi.org/10.1371/journal.pone.0029825.
Assaha, Dekoum V. M., Akihiro Ueda, Hirofumi Saneoka, Rashid Al-Yahyai, and Mahmoud W. Yaish. 2017. “The Role of Na+ and K+ Transporters in Salt Stress Adaptation in Glycophytes.” Frontiers in Physiology 8 (July): 509. https://doi.org/10.3389/fphys.2017.00509.
Bárzana, Gloria, and Micaela Carvajal. 2020. “Genetic Regulation of Water and Nutrient Transport in Water Stress Tolerance in Roots.” Journal of Biotechnology 324 (December): 134–42. https://doi.org/10.1016/j.jbiotec.2020.10.003.
Begum, Naheeda, Cheng Qin, Muhammad Abass Ahanger, Sajjad Raza, Muhammad Ishfaq Khan, Muhammad Ashraf, Nadeem Ahmed, and Lixin Zhang. 2019. “Role of Arbuscular Mycorrhizal Fungi in Plant Growth Regulation: Implications in Abiotic Stress Tolerance.” Frontiers in Plant Science 10. https://www.frontiersin.org/articles/10.3389/fpls.2019.01068.
Bonfante, Paola, and Andrea Genre. 2010. “Mechanisms Underlying Beneficial Plant–Fungus Interactions in Mycorrhizal Symbiosis.” Nature Communications 1 (1): 48. https://doi.org/10.1038/ncomms1046.
Bücking, Heike, Elliot Liepold, Prashant Ambilwade, Heike Bücking, Elliot Liepold, and Prashant Ambilwade. 2012. The Role of the Mycorrhizal Symbiosis in Nutrient Uptake of Plants and the Regulatory Mechanisms Underlying These Transport Processes. Plant Science. IntechOpen. https://doi.org/10.5772/52570.
Clausing, Simon, and Andrea Polle. 2020. “Mycorrhizal Phosphorus Efficiencies and Microbial Competition Drive Root P Uptake.” Frontiers in Forests and Global Change 3. https://www.frontiersin.org/articles/10.3389/ffgc.2020.00054.
Diagne, Nathalie, Mariama Ngom, Pape Ibrahima Djighaly, Dioumacor Fall, Valérie Hocher, and Sergio Svistoonoff. 2020. “Roles of Arbuscular Mycorrhizal Fungi on Plant Growth and Performance: Importance in Biotic and Abiotic Stressed Regulation.” Diversity 12 (10): 370. https://doi.org/10.3390/d12100370.
El-Sherbeny, T.M.S., Abeer M. Mousa, and El-Sayed R. El-Sayed. 2022. “Use of Mycorrhizal Fungi and Phosphorus Fertilization to Improve the Yield of Onion (Allium Cepa L.) Plant.” Saudi Journal of Biological Sciences 29 (1): 331–38. https://doi.org/10.1016/j.sjbs.2021.08.094.
“Fungal Extracellular Enzyme Activity.” 2023. In Wikipedia. https://en.wikipedia.org/w/index.php?title=Fungal_extracellular_enzyme_activity&oldid=1136267071.
Giambalvo, Dario, Gaetano Amato, Davide Borgia, Rosolino Ingraffia, Calogero Librici, Antonella Porto, Guglielmo Puccio, Paolo Ruisi, and Alfonso Frenda. 2022. “Nitrogen Availability Drives Mycorrhizal Effects on Wheat Growth, Nitrogen Uptake and Recovery under Salt Stress.” Agronomy 12 (November): 2823. https://doi.org/10.3390/agronomy12112823.
Glick, Bernard R., Zhenyu Cheng, Jennifer Czarny, and Jin Duan. 2007. “Promotion of Plant Growth by ACC Deaminase-Producing Soil Bacteria.” European Journal of Plant Pathology 119 (3): 329–39. https://doi.org/10.1007/s10658-007-9162-4.
Heijden, Marcel G. A. van der. 2010. “Mycorrhizal Fungi Reduce Nutrient Loss from Model Grassland Ecosystems.” Ecology 91 (4): 1163–71. https://doi.org/10.1890/09-0336.1.
Heijden, Marcel G. A. van der, Francis M. Martin, Marc-André Selosse, and Ian R. Sanders. 2015. “Mycorrhizal Ecology and Evolution: The Past, the Present, and the Future.” New Phytologist 205 (4): 1406–23. https://doi.org/10.1111/nph.13288.
Houser, Matthew, and Diana Stuart. 2020. “An Accelerating Treadmill and an Overlooked Contradiction in Industrial Agriculture: Climate Change and Nitrogen Fertilizer.” Journal of Agrarian Change 20 (2): 215–37. https://doi.org/10.1111/joac.12341.
Huey, Chew Jia, Subash C. B. Gopinath, M. N. A. Uda, Hanna Ilyani Zulhaimi, Mahmad Nor Jaafar, Farizul Hafiz Kasim, and Ahmad Radi Wan Yaakub. 2020. “Mycorrhiza: A Natural Resource Assists Plant Growth under Varied Soil Conditions.” 3 Biotech 10 (5): 204. https://doi.org/10.1007/s13205-020-02188-3.
Johnson, Nancy C., and Catherine A. Gehring. 2007. “CHAPTER 4 – Mycorrhizas: Symbiotic Mediators of Rhizosphere and Ecosystem Processes.” In The Rhizosphere, edited by Zoe G. Cardon and Julie L. Whitbeck, 73–100. Burlington: Academic Press. https://doi.org/10.1016/B978-012088775-0/50006-9.
Juniper, S., and L. K. Abbott. 2006. “Soil Salinity Delays Germination and Limits Growth of Hyphae from Propagules of Arbuscular Mycorrhizal Fungi.” Mycorrhiza 16 (5): 371–79. https://doi.org/10.1007/s00572-006-0046-9.
Lambers, Hans, Enrico Martinoia, and Michael Renton. 2015. “Plant Adaptations to Severely Phosphorus-Impoverished Soils.” Current Opinion in Plant Biology 25 (June): 23–31. https://doi.org/10.1016/j.pbi.2015.04.002.
Lang, Friederike, Jürgen Bauhus, Emmanuel Frossard, Eckhard George, Klaus Kaiser, Martin Kaupenjohann, Jaane Krüger, et al. 2016. “Phosphorus in Forest Ecosystems: New Insights from an Ecosystem Nutrition Perspective.” Journal of Plant Nutrition and Soil Science 179 (2): 129–35. https://doi.org/10.1002/jpln.201500541.
Li, Hui, Na Luo, Li Jun Zhang, Hai Ming Zhao, Yan Wen Li, Quan Ying Cai, Ming Hung Wong, and Ce Hui Mo. 2016. “Do Arbuscular Mycorrhizal Fungi Affect Cadmium Uptake Kinetics, Subcellular Distribution and Chemical Forms in Rice?” Science of The Total Environment 571 (November): 1183–90. https://doi.org/10.1016/j.scitotenv.2016.07.124.
Melissa. 2018. “Understanding Nitrogen Loss: Leaching.” ESN Smart Nitrogen (blog). September 17, 2018. https://smartnitrogen.com/smart-talk/understanding-nitrogen-leaching/.
Moghadam, Hamid Reza Tohidi. 2016. “Application of Super Absorbent Polymer and Ascorbic Acid to Mitigate Deleterious Effects of Cadmium in Wheat.” Pesquisa Agropecuária Tropical 46 (March): 09–18. https://doi.org/10.1590/1983-40632016v4638946.
Mollavali, Mohanna, Henrike Perner, Sascha Rohn, Peer Riehle, Franziska S. Hanschen, and Dietmar Schwarz. 2018. “Nitrogen Form and Mycorrhizal Inoculation Amount and Timing Affect Flavonol Biosynthesis in Onion (Allium Cepa L.).” Mycorrhiza 28 (1): 59–70. https://doi.org/10.1007/s00572-017-0799-3.
Morar, Florica, Laszlo Barna Iantovics, and Adrian Gligor. 2018. “Analysis of Phytoremediation Potential of Crop Plants in Industrial Heavy Metal Contaminated Soil in the Upper Mures River Basin.” Journal of Environmental Informatics 31 (March). https://doi.org/10.3808/jei.201700383.
Nichols, Kristine A. 2008. “Indirect Contributions of AM Fungi and Soil Aggregation to Plant Growth and Protection.” In Mycorrhizae: Sustainable Agriculture and Forestry, edited by Zaki Anwar Siddiqui, Mohd. Sayeed Akhtar, and Kazuyoshi Futai, 177–94. Dordrecht: Springer Netherlands. https://doi.org/10.1007/978-1-4020-8770-7_7.
Posta, Katalin, Nguyen Hong Duc, Katalin Posta, and Nguyen Hong Duc. 2019. Benefits of Arbuscular Mycorrhizal Fungi Application to Crop Production under Water Scarcity. Drought – Detection and Solutions. IntechOpen. https://doi.org/10.5772/intechopen.86595.
Read, D. J., and J. Perez-Moreno. 2003. “Mycorrhizas and Nutrient Cycling in Ecosystems – a Journey towards Relevance?” New Phytologist 157 (3): 475–92. https://doi.org/10.1046/j.1469-8137.2003.00704.x.
Riaz, Muhammad, Muhammad Kamran, Yizeng Fang, Qianqian Wang, Huayuan Cao, Guoling Yang, Lulu Deng, et al. 2021. “Arbuscular Mycorrhizal Fungi-Induced Mitigation of Heavy Metal Phytotoxicity in Metal Contaminated Soils: A Critical Review.” Journal of Hazardous Materials 402 (January): 123919. https://doi.org/10.1016/j.jhazmat.2020.123919.
Roser, Max, and Lucas Rodés-Guirao. 2013. “Future Population Growth.” Our World in Data, May. https://ourworldindata.org/future-population-growth.
Ruiz-Lozano, J. M., and R. Azcón. 1995. “Hyphal Contribution to Water Uptake in Mycorrhizal Plants as Affected by the Fungal Species and Water Status.” Physiologia Plantarum 95 (3): 472–78. https://doi.org/10.1111/j.1399-3054.1995.tb00865.x.
Selosse, Marc-André, Franck Richard, Xinhua He, and Suzanne W. Simard. 2006. “Mycorrhizal Networks: Des Liaisons Dangereuses?” Trends in Ecology & Evolution 21 (11): 621–28. https://doi.org/10.1016/j.tree.2006.07.003.
Shrivastava, Pooja, and Rajesh Kumar. 2015. “Soil Salinity: A Serious Environmental Issue and Plant Growth Promoting Bacteria as One of the Tools for Its Alleviation.” Saudi Journal of Biological Sciences 22 (2): 123–31. https://doi.org/10.1016/j.sjbs.2014.12.001.
Stanca, A. Michele, Ignacio Romagosa, Kazuyoshi Takeda, Tomas Lundborg, Valeria Terzi, and Luigi Cattivelli. 2003. “Chapter 9 – Diversity in Abiotic Stress Tolerances.” In Developments in Plant Genetics and Breeding, edited by Roland von Bothmer, Theo van Hintum, Helmut Knüpffer, and Kazuhiro Sato, 7:179–99. Diversity in Barley. Elsevier. https://doi.org/10.1016/S0168-7972(03)80011-7.
Takács, T., and I. Vörös. 2005. “Effect of Metal Non-Adapted Arbuscular Mycorrhizal Fungi on Cd, Ni and Zn Uptake by Ryegrass.” Acta Agronomica Hungarica 51 (3): 347–54. https://doi.org/10.1556/AAgr.51.2003.3.13.
Wang, Yuanpeng, Jing Huang, and Yanzheng Gao. 2012. “Arbuscular Mycorrhizal Colonization Alters Subcellular Distribution and Chemical Forms of Cadmium in Medicago Sativa L. and Resists Cadmium Toxicity.” PLOS ONE 7 (11): e48669. https://doi.org/10.1371/journal.pone.0048669.
“Water Acquisition.” n.d. Mycorrhizal Applications | Leaders in the Production of Mycorrhizal Fungi (blog). Accessed January 29, 2023. https://mycorrhizae.com/how-it-works/water-acquisition/.
Yahia, Elhadi M. 2019. “Chapter 1 – Introduction.” In Postharvest Physiology and Biochemistry of Fruits and Vegetables, edited by Elhadi M. Yahia, 1–17. Woodhead Publishing. https://doi.org/10.1016/B978-0-12-813278-4.00001-4.
Yan, An, Yamin Wang, Swee Ngin Tan, Mohamed Lokman Mohd Yusof, Subhadip Ghosh, and Zhong Chen. 2020. “Phytoremediation: A Promising Approach for Revegetation of Heavy Metal-Polluted Land.” Frontiers in Plant Science 11. https://www.frontiersin.org/articles/10.3389/fpls.2020.00359.
About the author
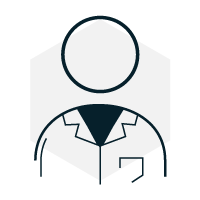
Madalyn Shen
Madalyn is a 10th grader at the Germantown Friends School. She loves learning about sustainable methods that people can apply to their everyday lives, especially in light of serious environmental problems. She has an aquaponics system in her backyard, which is a great way of growing organic vegetables and keeping her fish and turtle tank clean. Madalyn also plays tennis to alleviate stress.